7
Microbiomes and the Soil–Human Health Continuum
Given their well-established importance in maintaining the biochemistry inherent to health, there is strong motivation to include soil microorganisms, and other biota, in assessments of health from soils and humans. In fact, in soils today nearly a third of tests recommend microbial measurements of soil respiration, biomass, or nitrogen mineralization to characterize biological properties in assessment of health (Lehmann et al. 2020). Similarly, there is mounting evidence that the human microbiome plays an important role in human health (Turnbaugh et al. 2006; Lynch and Pedersen 2016). Given the higher resolution information on microbial content and processes provided by high-throughput sequencing technologies and mass spectrometric methods, microbiome-derived content could be untapped sentinels of soil and human health.
Microbes are likely to be among the key factors explaining how the environment affects human health. While there has been substantial work on understanding how the microbiome of the built environment can affect health (NASEM 2017; Gilbert et al. 2018), far less work has specifically linked soils and their microbiomes to human health outcomes. Most of the evidence linking soil health and human health by way of the microbiome focuses on direct ingestion of soil or contaminated food, aerosolized dust, and percutaneous transfer. Areas of interest in this research include the role of diet as modulated by the gut microbiome, toxins and pollutants derived from microbial metabolisms, and soil-derived pathogens or toxins on human health (see Chapters 3, 5, and 6). There is also indirect evidence that soil health may be tied to human health via the beneficial role of exposure to microbes in human development and the maintenance of health, notably on the immune, metabolic, and central nervous systems. Both exposure to environmental microbes and colonization of the human gut, oral cavity, lung, skin, and urogenital tract are essential for normal function of these organ systems (Thompson et al. 2017). The link between the soil microbiome and human health is particularly compelling because it offers the potential as a modifiable factor and health
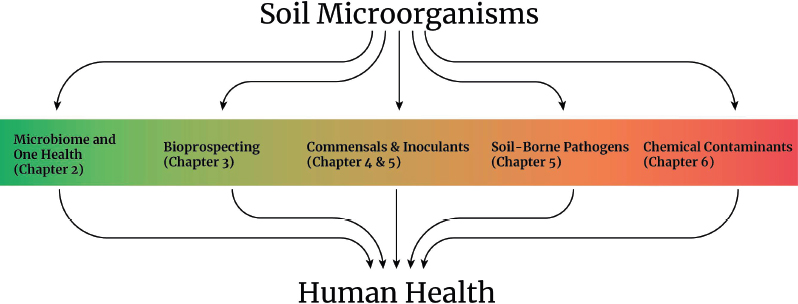
indicator that can reduce health inequities; this is in contrast to environmental and social determinants of health such as access to greenspace and biodiversity that require long-term investment to change.
This chapter focuses on the microbiomes of soils and humans and their relationship to health in each compartment (soils and humans) and across compartments. The focus is on the coordinated invisible ecosystem of microorganisms and less on specific microorganisms, as these are covered in other areas of this report (Figure 7-1). For instance, Chapter 2 introduces how the microbiome can be incorporated into a One Health framework, provides linkages between soil, plant, and animal systems, and includes a cross-system taxonomic analysis that highlights the shared and unique members (see Figure 2-9). Chapter 5 discusses the direct linkage between soil microbes and human health in their role as soil-borne pathogens (such as Escherichia coli, Clostridium tetani, and Coccidioides) and mycotoxins. Additionally, in Chapter 6, the effects of microbial transformations of soil chemical contaminants on health are discussed. Additional human health benefits conferred by soil microbiota, such as their ability to produce antibiotic and therapeutic agents (Chapter 3) or their roles as inoculants for soil management (Chapters 4 and 5) are also detailed in other chapters of the report.
Instead, this chapter offers a forward-looking perspective of the role for the microbiome in the soil–health continuum (Figure 7-2). The approach taken in this chapter underscores the identification of two primary knowledge gaps. Firstly, there is a pressing need to determine which microbial features, if any, contribute to quantifying or fortifying health in both human and soil systems. The ultimate goal for this knowledge is to leverage this understanding for the development of improved and rapid diagnostics or for new biotic-inspired therapeutics such as inoculants or probiotics. Secondly, there is a necessity to comprehend the direct and indirect roles of soil, alongside other environmental factors, in influencing human microbial colonization and subsequent
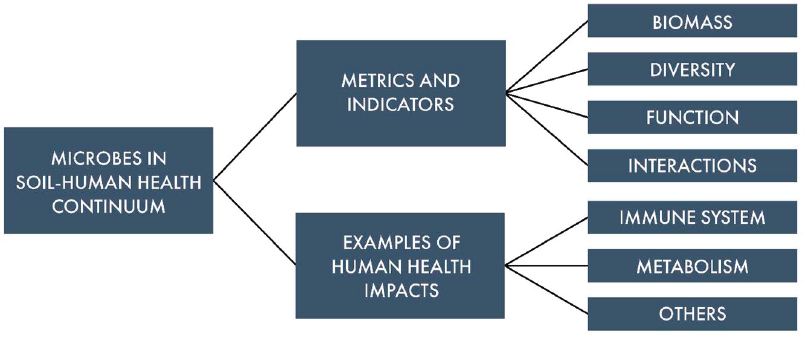
health outcomes. Such investigation involves delving into the relatively sparse or disconnected research regarding the microbiome continuum that links soil and human systems.
This chapter addresses two overarching research needs (Figure 7-2). The first section explores the current utilization of microbiome content to assess health status both in human and soil systems. This segment concludes with an examination of emerging technologies and analyses showing promise in identifying new microbiome-derived indicators for health or disease status in humans, crops, and soil systems. The second part of the chapter assesses the existing evidence regarding the microbiome continuum linking soil systems and human systems. It focuses on areas with the most compelling research evidence today: (1) the connection of environmental microorganisms to immune development and allergic disease and (2) the role of gut microbes in modifying or extracting nutrients from food for human health. In summary, by exploring the microbiome continuum from soils to human systems, the valuable insights that will inform diagnostics and interventions and promote health across both ecosystems can be unlocked.
MICROBIOME FEATURES AS INDICATORS OF HEALTH STATUS
Microbiomes as Modulators of Soil and Human Health
Structurally speaking, host systems such as the human gut microbiome and the plant rhizosphere (soils attached to or impacted by plant roots) exhibit numerous physical and chemical similarities. For example, both communities are partially shaped by host activity. In the human gut, microbial composition can be altered by dietary shifts, medication usage, host health status, and environmental factors, while the host also generates compounds such as mucin to nourish and facilitate microbial colonization (Fassarella et al. 2021). Similarly, microorganisms in the rhizosphere respond to
root exudation, where plants secrete metabolites, influencing microbial activity and community dynamics (Seitz et al. 2022). However, there are critical differences between these two habitats, as outlined below. Together these shared principles and unique drivers influence the way microbiomes are analyzed and collected and how they are interpreted in a health context.
Broadly, both gut and soil microbiomes provide benefits to their hosts through decomposition (e.g., fiber, proteins), nutrient extraction, and hormone production. As detailed later in this chapter, the human gut microbiota metabolizes proteins and fiber from the diet, generating short-chain fatty acids for the host energy. Similarly, soil microbiomes decompose litter and process metabolites from root exudation, shaping carbon and nitrogen pools crucial for maintaining soil biotic and abiotic structure as well as for plant health. In the gut microbiome, microbiota synthesize vitamins (e.g., vitamins B and K) and produce metabolic products that nurture human host cells (Nicholson et al. 2012). Similarly, soil microbiomes, particularly bacteria and fungi in the rhizosphere, play critical roles in extracting nutrients from soil and transferring them to host plants. Bacteria excrete compounds or enzymes that mobilize iron and other metals, acquire nutrients such as phosphorus and nitrogen, and produce phytohormones with regulatory properties for host plants (Chepsergon and Moleleki 2023). Additionally, mycorrhizal fungi can extend nutrient uptake beyond the plant roots by a factor of 1,000 (Larcher 1995) and alter phytohormone concentrations to promote drought tolerance (Bahadur et al. 2019). In return, the plants allocate a substantial amount of carbon to the underground fungal network, roughly equivalent to one-third of the annual carbon dioxide emissions from fossil fuels (Hawkins et al. 2023). Thus, gut and soil microbiomes play vital roles in hormone regulation and nutrient acquisition.
In both humans and rhizosphere soils, beyond providing nutritional benefits, the microbiome acts as a formidable barrier against the invasion and proliferation of pathogenic microorganisms (Nakatsuki et al. 2017; Zheng et al. 2020; Gu et al. 2022). In humans, microbiomes confer this resistance by induction of an immune response, competitive exclusion to make colonization inaccessible to other pathogens, production of antimicrobial substances, maintenance of intestinal barrier function, and immune training in early life (Zheng et al. 2020). In plants, commensal microbes can serve as a passive barrier by occupying niches that become inaccessible to pathogens, but current evidence also suggests that the plant immune response may be partially suppressed to allow for commensal colonization (Durán et al. 2018). Taken together, these findings highlight the complexity of microbial interactions in promoting host health and defense mechanisms, offering valuable insights for both human health and agricultural practices.
This report commonly refers to microbiomes associated with their broad habitat categories such as the gut and rhizosphere, but it is important to acknowledge that each habitat comprises vast microheterogeneity. Therefore, generalizing shared features may oversimplify the diverse metabolic regimes that exist in each compartment and span small physical distances or fluctuate over daily temporal scales. For instance, within the intestinal lumen, microbial density increases from the stomach to the colon, and there are distinct gradients of microbiota and metabolic lifestyles between the lumen and the adjacent mucus layer (Li et al. 2015). Similar nutrient gradients are observed between
the bulk soil and rhizosphere, and even within the rhizosphere, where specific taxonomic compositions and functionalities are associated with different microenvironments (Ling et al. 2022; Fitzpatrick et al. 2023).
The committee also recognizes that there are differences across gut and rhizosphere systems, which provide unique challenges in the microbiome methodologies and their analyses, a feature highlighted below in Table 7-1. For example, rhizosphere systems are external to their host and embedded within the environment, which means these microbiomes experience diurnal and seasonal fluctuations in temperature, nutrients, and water availability and are open to microbial dispersal. In contrast, the gut system is internal and is well modulated by the host in temperature and pH, with dispersals only happening via ingestion. These differences contribute to differences in the active and dormant members in each system, with proportionally more dormant cells in the soil and rhizosphere than in the gut (Lennon and Jones 2011), a finding that has ramifications for how the communities are composed, structured, and buffer perturbations. In summary, the committee has attempted to provide threads of comparison and context for identifying areas of synergy and perhaps knowledge transferability, while still recognizing the domain specifics that contribute to these unique microbiota, their derived processes, and host relationships across soil and human habitats.
Microbiomes as Diagnostic Agents for Health
Given the shared roles as modulators of chemical transformations and host-interactions in human and soil systems, the potential exists to use microbiome content as health indicators in soil, crop, and human systems. Because microbial catalysis is responsible (either directly or a few steps removed) for many of the chemical indices used today as health indicators, it is also believable that the microbiome functional content underpinning these transformations could serve as an even earlier indicator of health changes prior to measured chemical differences. In this scenario, microbiomes themselves may act as canaries in the coal mine, providing early warning signals for other desirable or undesirable outcomes (Gu et al. 2022). While the soil and human microbiomes have considerable potential to serve in this capacity, the ability to define and interpret microbial health indicators is limited today by sparse understanding of the ecology and function of microorganisms in both soil and human systems. Bridging this gap, along with experimental design and methodological advances for sampling and analyzing microbiome content along health states, will improve the incorporation of microbiome data in health monitoring (Wilhelm et al. 2023).
This section provides an inventory of microbiome features that are used to infer health in both human and soil systems and is organized based on different microbial units that have been used, or proposed to be used, as health indicators. The committee categorized the microbiome into four constituent classes that can be used to inform health status: (1) biomass, (2) compositional, (3) functional, and (4) interaction. Measurements derived from these framing categories can be used as indicators themselves (e.g., biomass) or used to calculate new indices (e.g., diversity metric) of health status in human, soil, and plant microbiomes. Table 7-1 summarizes a selection of these
Microbiota class types | Methods of measurement | Human systems | Soil and plant systems | Assumption | Caveats |
---|---|---|---|---|---|
Microbial biomass-Total mass of organisms per mass of sample [in use] | Chloroform fumigation, PLFA, SIR, fungal hyphal quantification, direct counts, qPCR, ergosterol (DeLuca et al. 2019). | Overall microbial biomass is not commonly estimated in human health assessments. | Bacterial and fungal biomass in soils is a commonly used metric of health (Ghimire et al. 2023). | In soils, greater biomass is commonly considered a positive health status. | (i) More biomass is not necessarily positive, as it does not provide information on which organisms are present (e.g., pathogen), (ii) biomass does not equate to increased rates of desired chemical reactions, (iii) high methodological variation in biomass estimation from soils. |
Microbial biomass-pathogen enumeration [in use] | Plate counts, direct counts, culturing, qPCR, 16S rRNA/ITS marker genes of relative biomass. | Isolated or identification of infectious agents using cultivation approaches (Washington 1996), growing appreciation for molecular diagnostics (Hodinka and Kaiser 2013). | Isolated or identification of infectious agents using molecular or cultivation approaches (Khiyami et al. 2014; Põlme et al. 2020; Hariharan and Prasannath 2021). | In host systems (mainly humans but also plant), greater pathogen loads are considered detrimental to health. | (i) Pathogen presence or abundance does not always equate to disease, (ii) other factors contribute to severity of disease (stress, wellness status, immunity). |
Targeted functional assays using known gene, enzyme, or process-based targets [in use] | Targeted approach (qPCR, qRT-PCR, enzyme assays, API) to quantify genes or enzymes that catalyze chemical reactions important to health in soils and humans. | API profiles and biochemical approaches and anti-microbial susceptibility to diagnose microorganisms associated with disease (Altheide 2020). | Acetylene reduction for nitrogen fixation, assay for potentially mineralizable nitrogen, or defined enzyme assays for carbon use are used in soil health assessments (Ferrocino et al. 2023). | Health status is specific to the enzyme selected, but increased activity is often considered a positive health outcome. | (i) Assays often have high internal variability cofounding interpretation, (ii) results can be impacted by methodology and environmental matrix (e.g., mineral content) that limit cross-study comparisons, (iii) requires a priori knowledge of the target, or health or disease agent, |
(iv) typically a bulk, single enzyme measurement that may not accurately represent the process. For example, beta-glucosidase enzyme activity may not accurately capture all soil carbon metabolism. | |||||
Microbial membership and abundance: alpha diversity [under development] | 16S rRNA/ITS rRNA gene (via amplicon sequencing or qPCR) provide information on microbial types/sample (richness) and their distribution (evenness) or can calculate diversity metrics per sample. Metagenomics is increasingly being used for compositional insights as well as functional diversity metrics (discussed below). | Decreased microbial richness of human gut microbiome was associated with increased disease metabolic markers (Le Chatelier et al. 2013). | Greater richness or diversity of mycorrhizal fungi and bacteria contribute to plant health; diverse soil communities have higher and more stable nutrient cycling (Fierer et al. 2021). | In both soils and human systems, increased species richness, evenness, and diversity metrics are thought to be associated with positive health outcomes. | (i) Not all DNA comes from intact or active cells (relic), (ii) relationships between diversity and health in both humans and soils are context dependent and not uniformly maintained across diverse hosts or sample types making interpretation challenging, (iii) greater richness from unwanted taxa may not contribute positively to health, (iv) sequencing technology may be a barrier to early adoption, requiring trained personnel to analyze and interpret microbiome information in health context. |
Microbiota class types | Methods of measurement | Human systems | Soil and plant systems | Assumption | Caveats |
---|---|---|---|---|---|
Microbial membership and abundance: beta diversity [under development] | Amplicon 16S rRNA/ITS rRNA provide taxonomic identity and relative abundance assess changes in beta diversity across samples or treatments. Metagenomics is increasingly being used for compositional content as well as functional beta diversity metrics (discussed below), e.g., how gene relative abundance patterns change with health status. | Microbiomes of disease patients fluctuate more than healthy patients, which can be associated with healthy plane (Brooks et al. 2017; Halfvarson et al. 2017). Core functional processes or members contribute to stability in face of changing conditions (Eisenstein 2020). | Greater abundance of mycorrhizae or plant beneficial microbes are associated with health (Fierer et al. 2021), some taxa inform lower health scoring soils. CST are associated with disease suppression in crops (Fujita et al. 2023). | Certain CSTs or microbiome membership will be associated with health, and these can be distinguished from unhealthy microbiomes. | (i) Not all DNA comes from intact or active cells (relic), (ii) multiple stable states exist in microbial communities without associated health change (Borton et al. 2023), (iii) overall community response or lack of response may mask changes in disease etiological agents, and (iv) sequencing technology may be a barrier to early adoption, requiring trained personnel to analyze and interpret microbiome information in health context. |
Untargeted functional gene diversity, content, or expression [under development] | Next-generation sequencing untargeted metagenomic, metatranscriptome, or metaproteome profiling of microbial communities. | Gene abundance and diversity predicted cardiovascular risk (Borton et al. 2023), functional shifts with inflammatory bowel disease (Lloyd-Price et al. 2019). | Genomic traits and environmental bioindicators of soil health were discovered (Wilhelm et al. 2023). | The relative abundance of genes or their expression or aggregated traits derived from this content can inform health status | (i) There is a small set of genes known today where biochemical knowledge that gene or gene product results in a directed health outcome, yet opportunity for discovery of new markers is desired, (ii) greater abundance of gene or its product does not dictate process rates, and (iii) this is an emerging technology with data difficult to analyze and interpret in health context today. |
Untargeted microbial derived metabolites diversity, content, or quantification [under development] | Targeted or untargeted - nuclear magnetic resonance, high-pressure liquid chromatography, or mass spectrometric based methods (GC-MS, LC-MS, FT-ICRMS) (Aderemi et al. 2021). | Specific metabolites can indicate disease, while profiles can be used to indicate wellness or carbon processing (Martinez et al. 2017). | Targeted phytohormone panels (Šimura et al. 2018) and untargeted metabolomics for soil quality functional assessment (Withers et al. 2020). | Microbial metabolism produces metabolites that can impact human, plant, and soil systems; these metabolites or suite of metabolites can be an indicator of health of system. | (i) Due to travel through the body or solubility/mobility through soils, measurement of the metabolite may be distant from production site, thus distorting signal interpretation, (ii) host and microbiome factors control metabolite conversions and consumption, such that metabolite may not be master regulator of disease, (iii) improved identification of metabolites and demonstration that they elicit health effect is needed, and (iv) newer technology with data difficult to analyze and interpret in health context. |
a API=analytical profile index; CST=community state types; FT-ICRMS=Fourier-transform ion cyclotron resonance mass spectrometry; GC-MS=gas chromatography–mass spectrometry; ITS=internal transcribed spacer; LC-MS= liquid chromatography–mass spectrometry; PLFA=phospholipid-derived fatty acids; qPCR=quantitative polymerase chain reaction; qRT-PCR=quantitative reverse transcription polymerase chain reaction; rRNA=ribosomal RNA; SIR=substrate-induced respiration.
SOURCE: Adapted from Fierer et al. (2021).
microbiome features that are used or proposed for use in diagnosing human and soil health conditions. The table outlines assumed relationships between the microbial measurement and health status and identifies some caveats with the application. Examples included in this table are not meant to be exhaustive, and they are organized first by microbiome measures that are actively used in clinical human diagnostics or soil health assessment frameworks today (as denoted by [in use]). Approaches under development today are indicated in the bottom half of the table. The interaction framework for health is nascent such that it is described in the text but not included in Table 7-1.
Discerning health metrics within and between the biological categories of biomass, composition, and function has relied on a variety of methods based on cultivation (e.g., colony forming units), molecular (e.g., quantitative PCR, amplicon sequencing), functional (e.g., enzyme and physiological assays), and -omics inspired approaches (e.g., metabolomics, metagenome, metatranscriptome, metaproteome). As such, Table 7-1 is not organized by method, as many of them measure different aspects of biomass, taxonomy, and functional components of the microbiome. For example, cultivation-based approaches can provide microbial load (biomass insights) as well as specific types of microorganisms (compositional insights). Similarly, both amplicon sequencing and metagenomics uncover the microbial membership and distribution in a sample. In the text that follows, the four microbiome constituents are described in subsections, articulating the importance of diversity metrics (composition), chemical catalysis (function), and food webs (interaction) as promising diagnostic indicators of ecosystem health and functioning, providing examples from soils to humans. This section is ultimately written to highlight microbiome indicators in use today but also highlight the promise of emerging technologies for deriving new microbiome-informed indicators. Although enthusiasm is universally shared across human and soil systems, the practice and quantifiable metrics of health using microbiome-derived information are not well defined at present.
Biomass Measurements of Health
Quantification or enumeration of biomass and its inferences to health varies across soils and human systems. In soil systems, microbial biomass, a measure of the mass of microbes both active and dormant per gram of soil, is a parameter often used to assess soil health. In fact, a third of soil health assessment tests, such as those from the European Commission or the Soil Management Assessment Framework, recommend microbial biomass measurements as a critical estimate of the biological properties of soils (Lehmann et al. 2020). This metric is estimated using a variety of methodologies, with lipid measurements or a chloroform fumigation-incubation being the most prescribed. Because microbial biomass accounts for a large proportion of total soil carbon and nitrogen, higher microbial biomass content or carbon is often considered as soil fertility constituent (Sparling 1997).
Fungal biomass is used as a biological indicator of soil health, as not only are these eukaryotic organisms vital to carbon and nutrient cycling but their hyphae and products play critical roles in improving soil structure and water retention. Typically, fungal biomass is assessed through lipid-based or ergosterol content or through microscopic
quantification of hyphal biomass. While conventionally managed soils are reported to exhibit decreased fungal biomass relative to more regenerative management strategies, a response thought to be due to tillage, high rates of fertilization, and fallow, this response is not always uniform (Frąc et al. 2018). In addition, the number of fungi versus bacteria in given soil, expressed as the fungal:bacterial ratio, has been historically used as an indicator of soil health, with higher ratios indicating a more sustainable soil system with higher carbon accrual. However, recently this ratio has received criticism as it is affected by methodological constraints that particularly impact fungal assessment in soils and it does not reflect current ecological understanding of complex, multi-trophic soil food webs (Fierer et al. 2021). For instance, some fungi and bacteria have overlapped or syntrophic functionalities in soils, which are functionalities that will not be captured by this ratio.
While biomass or its derivatives is one of the most used metrics for assessing soil health, the application of microbial biomass in health assessment has confounding interpretations. Recently, it has been debated whether biomass provides a useful, or readily interpretable, assessment of soil health (Fierer et al. 2021), as there are many biotic and abiotic factors that could contribute, directly or indirectly, to changes in soil microbial biomass. For instance, biomass does not account for the types or diversity of microbes present in a sample, such that overgrowth of a pathogen or single microbial type would increase overall biomass but would not necessarily be considered healthy. Additionally, more biomass does not necessarily equate with specific “healthy” catalytic properties of microorganisms. Despite these concerns, microbial biomass or microbial biomass carbon remains one of the commonly recommended biological-based indicators of healthy soils in use for soil health assessments.
Demonstrating how microbiome indicators are often ecosystem specific, broad measurements of microbial biomass were not commonly used as an indicator in host health in past human research. However, current work is increasingly recognizing the need for assessments of absolute abundance in addition to relative abundance of microbes in a microbiome. Specifically quantifying populations of microorganisms associated with disease, such as pathogen loads, is used to diagnose health in human and plant systems. Pathogen biomass can be enumerated using cultivation-based approaches to measure the amount of a specific type of microbe in sample mass or volume (e.g., selective media or most probable numbering techniques) as well as with molecular approaches that determine the abundance or relative abundance of a taxon in sample mass or volume (e.g., quantitative PCR and 16S or internally transcribed spacer region [ITS] ribosomal amplicon sequencing). Given that some taxa are obligately pathogenic, the presence of these members can be easily ascribed to poor health conditions observed in plants or human individuals. Yet, interpreting pathogen content is not always so clear-cut, as pathogen abundance does not necessarily correlate to disease severity (Swanson et al. 2007; Genin and Denny 2012; Leggett et al. 2012; Yadav and Pandey 2022). Further, many pathogens are opportunistic, meaning they can be present and active in healthy individuals, and disease severity is controlled instead by other conditions, such as stress or host immunity (Kaper et al. 2004). Thus, the biomass of targeted taxa is not always a robust indicator of health and depends on the taxon and the ecosystem.
Measuring Diversity and Composition of the Microbiome
Amplicon sequencing is a targeted approach that uses deep sequencing of a single gene to provide information on the diversity and composition of the microbiome. The sampling of bacteria and archaea (16S rRNA gene), fungi (ITS), and eukaryotes (18S rRNA gene) has provided new dimensions on the microbial community constructs that contribute to health across ecosystems. Data from amplicon sequencing projects can provide three lines of microbial information: (1) measurements of diversity such as richness (number of types of microbes in a sample) or evenness (their distribution in a sample), (2) the microbial composition (which microbes are present in a sample), which provides insights into taxa that are indicators of disease or health status (Wilhelm et al. 2022), and (3) the relative abundance of each member in a sample, which describes the distribution of microbial members (e.g., enrichment or dominance that can occur along a health axis).
Today, because of increased affordability and streamlined data analytics, there is widespread adoption of amplicon-based analyses of microbial communities across habitats from soil to plant and human microbiomes. This reduced cost per sample, and the ability to process hundreds to thousands of samples, allows researchers to better contextualize heterogeneity with a sample site, developing more robust temporal and spatial awareness of microbial diversity and membership dynamics. For example, it is now warranted to sample microbial communities during different temporal stages of soil management or crop and human development, both in health and disease, to capture monthly and yearly scale changes in the microbiome (Lauber et al. 2013) or even hourly responses to daily routines and fluctuations (e.g., temperature and feeding). Similarly, more intensive sampling of soil compartments (e.g., rhizoplane from rhizosphere) or anatomical sites (e.g., different sections of the gastrointestinal tract) will further discern microbiota responsive signals from natural variation (Singh et al. 2020; Chinda et al. 2022). Additionally, in soils, regional differences in climate and soil type, as well as local land management differences, can make comparisons of microbiome diagnostic patterns across studies or even samples within closely related plots confounding. Similarly, human genetic backgrounds, the history of clinical treatments, and variations in diets, age, and other factors confound universal microbiome metrics for human health (Falony et al. 2016). Amplicon sequencing today is an important tool for allowing researchers to sample this heterogeneity in microbiomes across different gradients of variation.
Metrics derived from amplicon sequencing, either organismal or diversity-based indicators, are being evaluated as diagnostic tools that could be incorporated into formalized health assessments in the near future. In this chapter, the committee focuses on compositionally defined diversity as an indicator of health as it is used in both soil and human microbiomes. Yet, the committee recognizes that diversity can be measured on any level of biological organization (Whittaker et al. 2001) and that microbiome diversity calculated using gene diversity, gene expression, or metabolite profiles may become more commonplace indicators of health status in the future as these methods have greater development and adoption as well (see the section “Functional Components of the Microbiome” below).
The explanation of how compositional diversity can relate to health is best understood through the lens of the insurance hypothesis. Originally proposed by Yachi and Loreau (1999), this hypothesis suggests that increasing biodiversity can insure ecosystems against functional declines in response to environmental perturbations (see also Box 3-1). This stability is achieved because different species respond differently to environmental fluctuations, but they can have overlapping functions within an ecosystem (i.e., functional redundancy). Therefore, greater species diversity increases the likelihood of maintained functionality in response to perturbations. With advancements in measuring functional diversity directly (through gene content or expression, see the discussion in the next section), it may no longer be necessary to be inferred from compositional richness, which may or may not be directly related to functional content at the scale required to withstand environmental perturbations.
Diversity measurements based on compositional data are often touted as indicators of health (Lozupone et al. 2012). In the human gut, the presence of a higher number of microbial members (richness) is inferred to make the microbial community more resistant to stressful events, such as antibiotic treatments (Lozupone et al. 2012). Moreover, it has also been shown that richer and more diverse microbiomes can guard against pathogen proliferation (Zheng et al. 2020; Bertola et al. 2021). On the other hand, decreased compositional diversity in the microbiome has been linked with chronic conditions such as obesity and type 2 diabetes, gastrointestinal diseases, and neurodegenerative diseases, among others (Cho and Blaser 2012; Fan and Pedersen 2021). Compositional diversity metrics may be used to assess health status more broadly. For example, gingivitis (gum inflammation) is associated with a lower diversity of the oral microbiome and the dominance of the pathogen Porphyromonas gingivalis (Hajishengallis et al. 2012). The findings suggest that microbiome compositional diversity could be an indicator of health in adult humans.
Microbial communities are structured by a few more dominant members followed by thousands of rare members. These rare members are thought to act as a seedbank, preserving genetic diversity until it is needed. Although not abundant, these rare members can become provisionally enriched under specific environmental conditions, maintaining ecosystem performance in the face of changing conditions (Shade et al. 2014; Jousset et al. 2017). It is regarded that increased microbial compositional diversity or richness, often due to a large number of rare taxa, contributes to higher nutrient-cycling rates (Fierer et al. 2021). Additionally, in the rhizosphere, as in humans, increased microbial (both fungal and bacterial) diversity is associated with pathogen suppression for crop wellness (Bollmann-Giolai et al. 2022; Ling et al. 2022). Conversely, heavy metal contamination has been associated with a decrease in bacteria, fungi, and protist diversity due to both a loss of rare members and the increased dominance of a few select taxa that can resist changes in the abiotic conditions (Qi et al. 2022). In fact, the four most common threats to soil microbial diversity are cited as intensive human exploitation, land use change, soil contamination, and climate change (Jeffery and Gardi 2010; Tibbett et al. 2020). Thus, as emphasized in other chapters of this report, there is an ever-increasing interest in maintaining soil microbial diversity to maintain soil functionality and health.
Beyond microbial compositional richness and diversity, the relative abundance and taxonomic identity of members can serve as indicators of health status. For example, in the vaginal microbiome, the genus Lactobacillus play a major role in maintaining female health—such that increased lactobacilli prevent against vaginal infection and reduce the risk of acquisition of HIV and sexually transmitted pathogens (Das Purkayastha et al. 2020). Despite these clear linkages in research publications, not enough is known about the vaginal microbiome and disease to be able to add lactobacilli probiotics to reliably shift in the direction of improved health (France et al. 2022). Also, large cohort studies of healthy and unhealthy individuals combined with machine-learning approaches are paving the way for discovering new microbial classifiers of health (Lee and Rho 2022). Despite compelling results from individual studies, further investigation is needed to reveal the roles of human microbiota in all body habitats in order to support the development of microbiome-based diagnoses and therapeutics (Hou et al. 2022).
Compared to the human microbiome, specific microbiome-derived indicators of health are less defined in soil systems. There are generally regarded plant beneficial microbes that are used as inoculants (see Chapters 4 and 5), but more extensive studies assessing the presence, plant colonization, and persistence of biostimulants in the rhizosphere are warranted. It is also unknown whether taxa extant in soils can respond to land management to serve as indicators of health. To investigate this possibility, a study surveyed more than 900 agricultural soils from diverse geographic locations and management types across the continental United States, with each soil ranked with a health score based on biological, physical, and chemical measurements encompassed by the Comprehensive Assessment of Soil Health (CASH) framework (Wilhelm et al. 2022). Researchers identified microbial taxa that differentiated soils with low and high health scores, and these new organismal indicators also predicted conventional metrics of soil health. Although it is a single study and not partitioned for regional or crop differences, the results indicate that, as with the human gut, microbiome compositional information obtained through amplicon sequencing could inform measures of health status.
However, in human and soil systems, using ribosomal RNA (rRNA) and ITS-based metrics for assessing health in microbiomes is not without constraints (Ames et al. 2017; Matchado et al. 2024). Firstly, these methods provide limited taxonomic resolution and cannot differentiate well-known pathogens (e.g., Salmonella typhi from E. coli), necessitating higher resolution for accurate identification of health-associated microbial taxa. Secondly, biases in data processing and reliance on incomplete databases can skew detection and interpretation of microbial groups in the microbiome. Additionally, while taxonomic composition may offer some limited insights into potential functional roles (Nguyen et al. 2016; Douglas et al. 2020), these methods do not fully represent the overall metabolic activity or functional potential of the microbiome. Moreover, using DNA as input material fails to distinguish active from dormant microbes or extracellular DNA (Lennon and Jones 2011; Carini et al. 2016), which are prevalent in soils and the human gut and could further overrepresent inactive members. Understanding the distinction between active and dormant microbiota, along with their functional capacities, may become crucial indicators when assessing implications for soil and human health.
While rRNA and ITS sequencing provide valuable insights into microbial community composition, diversity, and dynamics, their use in health assessment could be bolstered with other methods discussed below and careful consideration of their limitations.
Functional Components of the Microbiome: A Promising Indicator of Health
Assessing metabolic potential There is broad recognition that harnessing microbiomes for improving plant, human, and soil health requires decoding the genetic underpinnings of the microbiome and how these molecular constituents are translated into chemical changes and ecosystem outputs. This is no trivial task, but newer -omics technologies, such as metagenomic sequencing, and other multi-omic technologies, such as metatranscriptomics, metaproteomics, and metabolomics, can provide advanced functional insights into the microbiome (Figure 7-3). This section defines these meth-
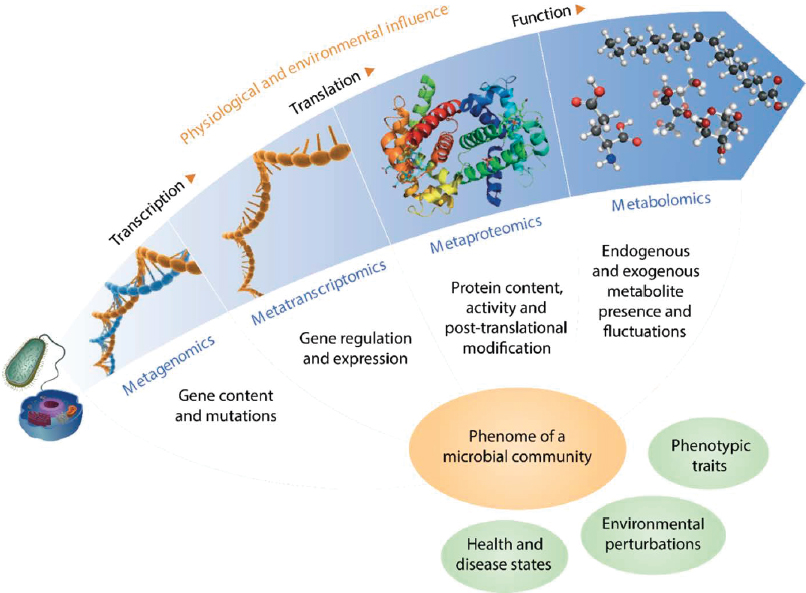
SOURCE: Used with permission of Springer Nature BV, from “A Multi-omic Future for Microbiome Studies”, Jansson and Baker, Nature Microbiology 1 (5), 2016; permission conveyed through Copyright Clearance Center, Inc.
ods and begins to illuminate how these tools and their measurement of the functional aspects of the microbiome may be called upon to assess health status in human and soil systems in the future.
It is increasingly possible to use untargeted sequencing approaches to inventory the cache of gene content in a microbial community, an approach known as metagenomics (Jansson and Baker 2016). Metagenomics approaches are best developed for bacteria and viruses (Kang et al. 2017; Emerson et al. 2018; Leleiwi et al. 2023), where results have showed greater ability to predict ecosystem outputs and health status. These methods are still in development for eukaryotic members like fungi and protists (Donovan et al. 2018). Instead of community-wide genomics (metagenomics) for eukaryotes, valuable insights into the functional attributes or traits of fungi have been gleaned from genomic approaches that sequence specific taxa individually or map environmental sequences to genomes derived from relevant isolates (Treseder and Lennon 2015; Ravn et al. 2021; Zhang et al. 2023). Microbial gene information derived from genomic sequencing not only yields taxonomic content (although not as deeply sampled as amplicon-based sequencing) but also provides critical measurements on the genes that govern metabolic or functional capabilities in a microbiome.
Specifically, metagenomics has advanced knowledge of human health, uncovering new metabolic assignments for previously uncultivated lineages (Wrighton et al. 2012; Di Rienzi et al. 2013), identifying potential for microbially derived antibiotics and secondary metabolites with health-promoting benefits (Crits-Christoph et al. 2018), and outlining the metabolic wiring underpinning the functional outputs critical for soil and human health (Table 7-1). Advances in data processing (software), decreased sequencing cost, and improvement of databases for data processing (Pasolli et al. 2019) have made it possible to apply metagenomics to larger cohort sizes and to utilize sequencing more effectively within a sample. The metagenomic-derived functional gene profiles derived from fecal microbial genomic content can predict human heart disease (Borton et al. 2023) and various types of cancers, from colon to prostate (Banerjee et al. 2015; Gao et al. 2022). Additionally, metagenomics can rapidly identify infectious disease-causing pathogens, such as bacteria, viruses, and fungi in a single test without the need for culturing (d’Humières et al. 2021), thereby playing a promising role in the clinical diagnoses of disease (Box 7-1). Yet, to date, there remain few FDA-approved microbial therapies (Jain et al. 2023).
In comparison to human clinical assessments, metagenomic studies have not been used to study soil health as often (Duque Zapata et al. 2023), and larger cohort studies with paired genomic insights and robust physical, chemical, and biological soil health indicators are needed. To these ends, genome-resolved metagenomic databases from soils are on the rise both in the public (Woodcroft et al. 2018) and private sectors,1 however, developing an open, collective database infrastructure, such as the Human Microbiome Project, and targeting agricultural soils with paired soil health data
___________________
1 See, for example, “Pattern Ag Announces World’s Largest Metagenomics Database,” September 18, 2023. Accessed April 30, 2024. https://www.pattern.ag/news/pattern-ag-announces-worlds-largest-metagenomics-database.
BOX 7-1
Human Microbiome Project
In 2007, the National Institutes of Health, through its Common Fund, established the Human Microbiome Project. In its first phase, the project used 16S rRNA sequencing to characterize microbial communities in the human body and metagenomic sequencing to learn about microbiome functions. In Phase II of the project, studies that focused on pregnancy and preterm birth, inflammatory bowel diseases, and stressors affecting individuals with prediabetes began to elucidate mechanisms of host–microbiome interactions (HMP 2019).
This novel undertaking spurred taxonomic sequencing and eventually molecular profiling of microbial gene content from the major ecological niches of the human body. Research has expanded from a focus on the gut microbiome to touch on many diverse sites of microbial colonization including oral, respiratory, vaginal, cutaneous, and ocular microbes. It has been found that a combination of an individual’s genetic background and current state of health, the molecular function and personalized strain-specific makeup, and environmental factors are all important predictors of a range of health outcomes. For example, a recent study profiled the bacterial composition, function, antibiotic resistance, and virulence factors in the gut microbiomes from a three-generation Dutch cohort and found that a larger proportion of variation in the gut microbiome is associated with cohabitation and environment than genetic relatedness (Gacesa et al. 2022). These large-scale studies, sampling thousands of humans over time and assessing the microbiome at different levels of biological units from composition to functional attributes, as well as their individual and coordinated relationship to health, can provide inspiration for the kind of research needed in soil and human systems.
specifically, could advance the discovery of microbial gene indicators in these soils. These efforts are buoyed by additional large-scale global soil microbiome catalogs, such as the Earth Microbiome Project (Thompson et al. 2017) and the federally funded Genomic Encyclopedia of Bacteria and Archaea project (Whitman et al. 2015). Yet, more studies are needed across geographic and agricultural landscapes with paired microbiome and soil health measurements (Wilhelm et al. 2022) to begin fortifying the predictive capabilities of the microbiome for soil health and fertility. The federal government has taken steps to develop the necessary infrastructure for sharing data across projects with a project called the National Microbiome Data Collaborative, which supports a Findable, Accessible, Interoperable, and Reusable (FAIR) microbiome data sharing network, through infrastructure, data standards, and community building (NMDC 2022).
However, reusing microbiome data collected across many different studies for machine-learning approaches (Box 7-2), especially those spanning analyses across soil
BOX 7-2
Machine Learning and Artificial Intelligence for the Microbiome
With the tremendous progress in multi-omics approaches and the popularity of their adoption, both the type and amount of data are increasing at an astronomical pace. Consequently, data wrangling, storing, and management have become an important part of microbiome projects as well as long-term information archiving. Data processing using artificial intelligence tools is also becoming popular in microbiome science. One such tool that has seen a surge in use is machine learning, which is a flexible set of tools that can tackle large datasets to identify, group, and predict patterns (Hernández Medina et al. 2022). Machine learning can be broadly classified into two groups: unsupervised machine learning and supervised machine learning. Unsupervised machine learning attempts to group observations and identifies major structures in a new dataset when a priori information or hypotheses are not available. On the other hand, supervised machine learning attempts to generate predictive models from training data when answers are known and a priori hypotheses are available (Asnicar et al. 2023). Deep learning is also a type of machine-learning tool that utilizes artificial neural network architectures. The networks are composed of nodes or neurons, which are essentially functions that can perform operations and forward the results to other nodes.
In recent years, machine learning has been applied to a wide range of microbiome questions, including assessing the complex physical and chemical interactions between the microbiome components, selecting microbiome features such as the abundance and distribution of biomarkers, predicting an environmental or host phenotype, and identifying changes in the microbiome composition (Hernández Medina et al. 2022). For human microbiomes, machine-learning tools are assisting clinicians with accurate prognosis and diagnosis in precision medicine. For example, Calderone et al. (2015) used supervised machine learning on a large dataset
and human compartments, is not trivial today. Barriers include that the data are often housed without metadata standards, hindering reprocessing of the data to create uniform analysis metrics. Public microbiome data are also not housed with health outcome data, and the latter is often linked within studies rather than housed in public repositories. Beyond data organization, while many artificial intelligence approaches may uncover genes or sets of genes linked to disease, many times these “predictors” lack accurate biochemical annotations with unknown functional outcomes. Thus, both from a data-mining point of view and a biochemical knowledge perspective, empowering gene content into health frameworks will require ongoing research. Yet, there are enough promising studies, especially on the human microbiome side, highlighting the promise that this content can be used for microbiome-inspired diagnostics and interventions.
Activity assessment of the microbiome
While metagenomics provides insights into the gene-encoded metabolic potential of a microbial community, it may not fully
comprising 2,357 human proteins and 453 viral proteins and predicted interactions between human proteins and viral proteins with up to 80-percent accuracy. Another study employed machine learning and cross-validation on 969 fecal metagenomes to predict colorectal cancer with over 80-percent accuracy (Thomas et al. 2019). Recently, Weis et al. (2022) used a clinical dataset comprising 300,000 mass spectra with 750,000 antimicrobial resistance phenotypes to detect resistance of potent pathogens such as Escherichia coli, Klebsiella pneumoniae, and Staphylococcus aureus. Machine-learning tools have also been used to predict pancreatic cancer using fecal microbiome signatures, determine the success of fecal transplantation, and assess the mortality risks in melanoma patients (Asnicar et al. 2023).
Soil microbiome studies are also utilizing different machine-learning algorithms. One of those algorithms that is routinely used is random forest analysis, which has detected macroecological patterns of soil bacteria (Ramirez et al. 2018), identified microbial predictors of soil multifunctionality (Delgado-Baquerizo et al. 2016b), predicted keystone taxa (Trivedi et al. 2017), identified global soil microbial carbon, predicted assembly of seedling microbiomes (Walsh et al. 2021), and found drivers of soil microbiome complexity (Yang et al. 2023). Fundamental soil science studies are also utilizing random forest analysis to predict soil parental materials (Heung et al. 2014), soil carbon stocks (Wiesmeier et al. 2011), soil texture (Chagas et al. 2016), soil respiration (Delgado-Baquerizo et al. 2016a), and optimal soil health parameters (Wilhelm et al. 2022).
While artificial intelligence tools have been adopted and implemented in human health and soil health studies separately, the linkage between the two health components has yet to leverage such efforts. The stage is indeed set for such exploration; however, enhanced data integration and interoperability will facilitate these linkages. Given that biochemical and microbiome signatures of health can be “nuanced” and challenging to distill from the deluge of data, the aforementioned tools can be particularly useful in establishing hypotheses for further investigation.
capture the dynamic responses to environmental perturbations (Jansson and Baker 2016). Instead, functional assays, which measure or attempt to measure active processes in the microbiome, may be more direct indicators of health. These can include either (1) targeted approaches that use process-based assays or (2) untargeted approaches that measure expressed gene products, such as transcripts or enzymes. In soil health assessments today, enzyme assays are commonly used as biological indicators. For example, beta-glucosidase and cellobiohydrolase assays are employed to estimate carbon decomposition activity in the Soil Management Assessment Framework (Stott et al. 2010), an assessment tool to evaluate impacts of land management on soil quality. While these process-based assays provide valuable insights into targeted processes like nitrification or potentially mineralizable soil nitrogen, their interpretation may be complicated by soil matrix effects and the inability to effectively capture all relevant processes, and the need for further validation persists (see Table 7-1 for a detailed discussion).
Untargeted approaches, such as metatranscriptomics (the sequencing of the transcribed cellular content in a sample) and metaproteomics (mass spectrometric analysis of proteins), sample the expressed gene content in soil and human microbiomes (Aguiar-Pulido et al. 2016). These methods offer opportunities to identify new, more comprehensive targets beyond those represented in the handful of established enzyme assays today, but they also can be more responsive than metagenome methods (or other DNA-based approaches) because they reveal an immediate response to perturbations (e.g., land management, diet interventions, disease onset). In both human and soil systems, metatranscriptomics and metaproteomics are technological advancements that could be leveraged for measuring and monitoring health aspects, offering new directions for research and clinical diagnosis and management (Berg et al. 2020; Bertola et al. 2021). While promising and growing in their application in soil (McGivern et al. 2021; Starke et al. 2021) and human microbiomes (Long et al. 2020; Borton et al. 2023; Wang et al. 2023), these methods are often only as robust as their underlying database used to contextualize the expression data, which can be challenging in samples with high strain diversity. The data collection can be affected by the heterogeneity of the matrix, and, compared to other methods, the cost to process and analyze at scale to identify health outcomes is limiting (Issa Isaac et al. 2019). These or other methods, such as quantitative stable isotope probing (Hungate et al. 2015; Wilhelm et al. 2021), probe-based functional profiling (Whidbey and Wright 2019), or flow cytometric single cell metabolic assays (Salazar et al. 2019), may further quantify the abundance of active and dormant microbes and processes in the future, providing better descriptions of microbial traits relevant to soil and human health outcomes.
Likewise, metabolomics—the study of low-molecular-weight organic compounds—can be targeted (directed at specific chemical compounds) or untargeted (simultaneous measurement of large number of compounds in a sample). This approach offers insight into the metabolic status of a sample, measuring the chemical products of the biological community. In humans, the detection of metabolites produced by gut microbes from dietary metabolism has been linked to pathologies such as hypertension, atherosclerosis, heart failure, obesity, kidney disease, and type 2 diabetes (German et al. 2005; Tang et al. 2019). In soil systems, metabolite approaches are used but trail studies in humans (Ellenbogen et al. 2024; Song et al. 2024) and are less developed for measuring health status. However, a recent study using nine different topsoils along a land use gradient with paired chemical and physical soil health data showed that the untargeted detection of more than 400 soil metabolites had discriminatory power as a potential soil quality indicator (Withers et al. 2020). Additionally, measurements of soil metabolomes from 188 backyard soils across 14 U.S. states demonstrated that soil metabolomes reflected the effects of local factors such as temperature, light level, and human activities on the soil (Nguyen et al. 2020). However, methodological improvements, including overcoming extraction biases from matrices, enhanced annotation and identification of soil metabolites, and discerning the fate of metabolites due to transient fluxes, will be necessary to identify the close relationships between microbiota and metabolomes in a wellness context from complex microbiomes in soils and the human gut (Song et al. 2024).
Both in human and soil microbiomes, multi-omics or the complementary use of multiple methods (metabolite, proteomic, genomic, transcriptomic approaches, see Figure 7-3) can provide more definitive evidence on the microbial metabolic pathways related to health status. The next step is to establish metrics to evaluate performance aspects of metabolomic and other multi-omic methods under a wide range of health and disease conditions, as well as management regimes, so that they can be used for the quantitative assessment of human health or soil health (Withers et al. 2020).
Interactions: An Unstudied Metric of Health
Biodiversity includes not only the number and type of species and their abundances but also the complex interactions among different species. There is growing appreciation for network-based analyses that incorporate taxonomic (co-association or abundance network) or functional (co-expression network) content to estimate the interactions that occur among different species and their contributions to ecosystem functioning (Harvey et al. 2017; Wagg et al. 2019; Box 7-3). It should be noted that these networks do not provide explicit evidence for species interactions but suggest that organisms or the gene content co-occur over space and time, hinting at a possible interaction potential. Networks constructed from taxonomic or functional information can be assessed for their connectivity, network size and structure, and relationship to health or ecosystem properties (Shi et al. 2016; Banerjee et al. 2019; Dundore-Arias et al. 2023). It is hypothesized that more complex and connected networks are more robust to disease and other biotic perturbations as well as abiotic stressors (Barabási et al. 2011). In support of this, a recent study of bacterial rhizosphere networks in tobacco plants strongly affected by the pathogen Ralstonia showed that disease-suppressive soils had greater network complexity and that the bacterial abundances of highly connected keystone taxa within disease-suppressive soils were negatively correlated with pathogen density (Zheng et al. 2021). Similar network approaches have been used in human gut microbiomes to identify coordinated species and pathways associated with inflammatory bowel disease that are absent in obese and healthy population cohorts (Chen et al. 2020). While nascent, network analyses that use culturing and multi-omics approaches to capture the interactions of phenotypes could be indicators of health in the future.
Building on the ideas of trophic structure comprising all soil biota, not just viral, archaeal, bacterial, or fungal members, it is increasingly apparent that biodiversity maintenance is an essential constituent for soil health. Here, the idea in soils is that preserving belowground biota from microbes to protozoan to animals such as nematodes and earthworms is critical not only for soil functioning today but also for long-term biodiversity management with repercussions extending to human health. Collectively, a loss of soil diversity (microbes, fungi, protozoa, and fauna) has been associated with lower plant diversity and crop yields, leading to reduced food security, erosion that reduces air and water quality, and increased soil-borne pathogen and pest load, which all can have indirect and direct human health impacts (Wall et al. 2015). Consequently, current research initiatives across global organizations, such as the Global Soil Biodiversity Initiative, are committed to inventorying and maintaining soil biodiversity to protect
BOX 7-3
Viruses
Viruses are key members of Earth’s microbiomes, shaping microbial community composition and metabolism. There is growing interest that bacteriophages that infect bacteria may be additionally sentinels of health in soil, cropping, and human microbiomes. This perspective is spurred by the fact that in the past decade the field of viral ecology has moved from quantifying virus-like particles in different environments to community-wide measurements, where sequencing the DNA or RNA from a sample (using metagenome or metatranscriptomes) offers the capacity to sample the genomic diversity of viruses living in soils, plant, and human systems (Sharma et al. 2021; Bhagchandani et al. 2023; Ma et al. 2024). These studies have reported that viruses are highly diverse and very abundant, infect a significant portion of their microbial host communities, and influence major biogeochemical processes mediated by microbes (Roux and Emerson 2022). Across human and terrestrial microbiomes, correlations between viral abundances and ecosystem services (e.g., climate-relevant biogeochemical indices [Emerson et al. 2018; Rodríguez-Ramos et al. 2022; Jansson and Wu 2023]), agricultural practices (Santos-Medellin et al. 2021; Liao et al. 2022), and human health lifestyle, status, or outcomes (Gregory et al. 2018; Chen et al. 2023) are often more informative than bacterial correlates alone, suggesting that further interrogation of viral communities can yield a more comprehensive understanding of microbiome functional networks and ecosystem processes. Thus, understanding the role of phages offers a promising avenue for predicting and maintaining health in both human and soil ecosystems.
soil and human health. Beyond microbial biomass, taxonomic, and functional metrics, soil biodiversity management should target the ecological complexity and food web architectures necessary to provide robustness of soil ecosystem services. In summary, an ecosystem management approach to health in soils can be extended to managing humans and plant health more holistically. As stipulated in the One Health framework, there is a recognized need to move beyond managing a single aspect of health, with biodiversity stewardship and overall ecosystem wellness being increasingly realized as a metric unto its own (FAO, UNEP, WHO, and WOAH 2022).
While promising, most of the modern -omics-based metrics are exploratory and have not been readily incorporated into human clinical or soil health assessments (Table 7-1). Similarly, interactions as a measurable unit of health is a newer concept not widely adopted today. Given the complexity of microbiomes, the reality of creating a robust, scalable health index using a single microbiome measurement (e.g., gene, organism, metabolite, interaction network) is unlikely. This situation is not entirely
different from applications of health assessments in humans and soils today, which use a handful of metrics derived from physical, biological, and chemical measures for a more comprehensive picture of wellness. To discover new indicators or features of the microbiome that can predict health in humans and soils (see Box 7-2), enhancing the spatial and temporal resolution of the data stored in collections, moving beyond a handful of 10–100 samples per study to thousands, will help resolve localized responsive “signals” across a background of heterogenous “noise.” Additionally, these analyses need to be performed when there is a clear health outcome or gradient to compare to, to detect capacity of microbiome-derived indicators to predict different health status. Artificial intelligence approaches will likely play a critical role in this discovery given the deluge of data produced from modern -omics methods. Rather than being derived from genes or sets of gene content, it is likely that microbiome functional estimates of health will be distilled to aggregate properties that capture more responsive indicators that may function as a final single health index (Lehmann et al. 2020).
SOILS, MICROBES, AND HUMAN HEALTH
Though microbes are often associated with disease and infection, the relationship between microbes and human health is far more complex. Research over the past few decades has unveiled the indispensable role of exposure to microbes in human development and the maintenance of health, notably on the immune, metabolic, and central nervous systems. Both exposure to environmental microbes and colonization of the human gut, oral cavity, lung, skin, and urogenital tracts appear to be important for normal function and protection against pathogenic microorganism and toxins (Thompson et al. 2017). Microbes outnumber human cells in the body, and their metabolic products make up over a third of the small molecules in the peripheral blood, many of which affect physiology (Wikoff et al. 2009).
To what extent exposure specifically to the soil microbiome influences human health is as yet unknown. Despite the growing capacity to characterize taxa and study the functions of microbiomes in humans and other systems through next-generation sequencing, the full continuum between soil health and human health by way of microbiome influence is not established in the literature. Additionally, even when skipping past the soil microbiome for which the metrics are under development, the committee found limited research that spans the investigation on how soil health more broadly may influence the nutrient density of foods in a way that would affect the gut microbiome’s metabolic capacity or human health (Chapter 5). Further, the committee found inconclusive support for the direct linkage of soil microbiomes to human gut microbiomes leading to human health outcomes. As such, this section focuses on areas where promising linkages have been made for (1) environmental microbes as immunomodulators for human health, (2) soil microbiomes and gut microbiomes in animal models, and (3) the gut microbiome as a connector between nutritional inputs and human health.
The Hygiene Hypothesis and the Importance of Balancing “Good” and “Bad” Microbial Exposures
Humans, like all eukaryotic life forms, have evolved from microbes and have co-evolved to form a symbiotic relationship with microbes (Domazet-Lošo and Tautz 2008). For example, the immune system is dependent on receiving appropriate inputs from microbial interactions, and these must be received early in life and then maintained and updated throughout life (Bach 2018; Rook 2021). It has been shown that maternal and familial transfer of microbiota is crucial for the development of an infant’s microbiota and that lifestyle factors that reduce this transfer and correlate with increased immunoregulatory disorders include caesarean deliveries, lack of breast feeding, antibiotic use, and diet (Penders and van Best 2022). Environmental exposures, including proximity to green space and farms, has also repeatedly been shown to be influential (von Mutius 2021). For example, Hanski et al. (2012) showed that environmental biodiversity based on land use data was correlated with a greater diversity of commensal skin bacteria commonly found in soil and vegetation, which translated into functional measurements of serum immune markers and lower rates of allergic disease.
Exposure to the environment and microbes is essential to the selection of a repertoire of cells that can eliminate pathogens while tolerating the microbiota and avoiding self-recognition and autoimmunity. Therefore, each individual develops an immune repertoire that is matched to the microbial world into which he or she is born and resides (Rook 2021, 2022). The individualization of this process has important implications for health. For example, purposeful infection with soil-transmitted helminths2 has shown some efficacy in early-stage trials for treating autoimmune diseases in humans, but later-stage clinical trials have not confirmed these results (Ryan et al. 2020). It may be that the elimination of helminth exposure during childhood in some parts of the world plays a role in the response (or lack thereof) to exposure to helminths later in life to treat autoimmune diseases (Rook et al. 2015).
The hygiene hypothesis, first proposed in the late 1980s based on data showing an inverse correlation between hay fever and the number of older siblings, suggests that reduced exposure to infections and microbes during childhood may contribute to the increasing prevalence of allergic and autoimmune diseases in developed countries (Strachan 1989). The hypothesis, popularized by the media, highlights the paradox that while hygiene is needed to avoid exposure to dangerous pathogens, humans also need exposure to “beneficial” microbes. Rook (2022) suggests a framework for reconciling these conflicting needs, as illustrated in Figure 7-4.
A common interpretation of the hygiene hypothesis is that humans have become “too clean for our own good.” Early humans lived in shelters built from natural products that were likely to provide beneficial microbial exposures. In contrast, modern homes
___________________
2 Helminths are worm-like parasites, such as flukes, tapeworms, and roundworms. Hookworm is an example of soil-transmitted helminth for which infection was common in the southern United States until eliminated by a public health campaign in the early 20th century. Worldwide, hookworm continues to cause hundreds of millions of infections in humans each year.
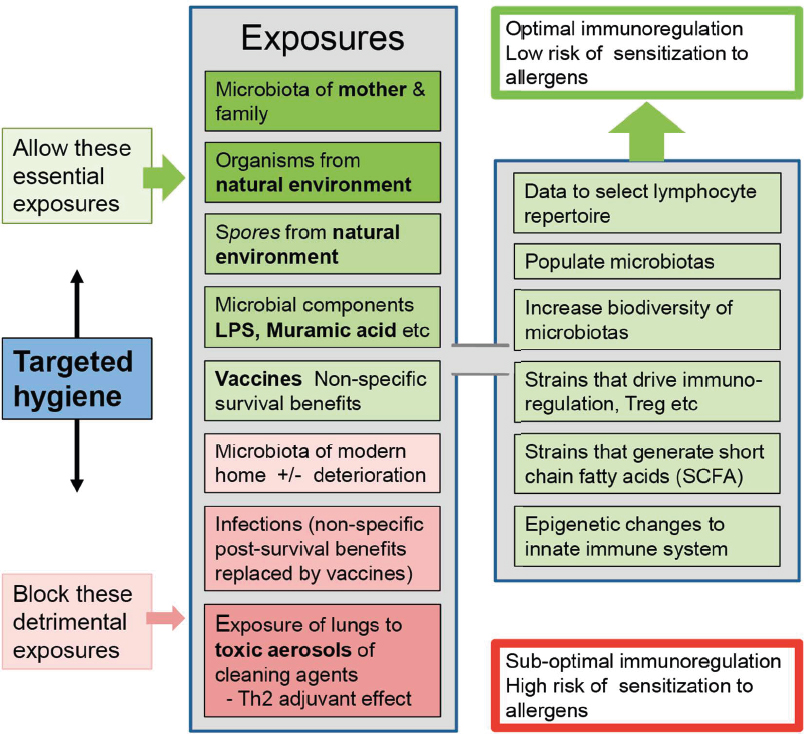
NOTES: The essential exposures include the microbiota of mothers, family, and the natural environment. Some of the benefits derive from molecular signals rather than colonization. The nonspecific immune system-training benefits of infections (assuming survival) can be replaced by vaccines. Targeted hygiene can maintain the essential exposure while protecting from pathogens.
SOURCE: Used with permission of Springer Nature BV, from “Human Evolution, Microorganisms, Socioeconomic Status and Reconciling Necessary Microbial Exposures with Essential Hygiene,” in Evolution, Biodiversity and a Reassessment of the Hygiene Hypothesis, Rook, 89, 2022; permission conveyed through Copyright Clearance Center, Inc.
are largely built from synthetic products, and bacterial and fungal microbiota frequently invade damp and deteriorating modern homes and can produce infections (Rook and Bloomfield 2021). Therefore, upkeep and cleanliness are important. However, this hygiene must be balanced against the risks of exposure to cleaning agents that may have immunostimulatory properties. Exposure to antigens via sites like the gut, airways, or skin in the presence of toxins, common in cleaning products, activate TH2 immune responses and can result in allergies and autoimmune disease (Akdis 2021; Rook and Bloomfield 2021). Therefore, both building products and household cleaners are likely to influence escalating rates of allergic and autoimmune disease.
Another common misconception is that childhood infections are necessary to “strengthen” the immune system. Today’s common infections of childhood are mostly crowd infections that were not present during most of human evolution, and the risks of infection likely outweigh any potential benefits of long-term and nonspecific immunity (Rook and Bloomfield 2021). Moreover, many infections can now largely be replaced by vaccines, which have been shown to have disease-specific benefits and to improve resistance to other infectious agents (Dagenais et al. 2023).
Putative Role for Soils as Influencers of Gut Microbiomes
From animal models, there is evidence that soil biodiversity is interrelated with the mammalian gut microbiome. For example, a study assessing the impact of different lifestyles on the development of mouse gut microbiomes found that mice in contact with soils and dust harbored a gut microbiome with greater diversity and richness (Zhou et al. 2016). While this diversity was not correlated to nutritional performance or overall health, it was found that these mice had lower serum immunoglobulin. Such results indicate that contact with soils could enhance gut microbial diversity and innate immunity, in support of the hygiene hypothesis by way of the gut microbiome and soils. Supporting the stimulatory role of soil biodiversity for gut microbiome diversity, another study found that gut microbial diversity increased in mice that were in contact with non-sterile soil, while it was unaffected when the mice were in contact with sterile soil (Zhou et al. 2018). The study also showed that non-sterile soils were a key factor influencing the gut microbiota and its effect was comparable to the effect caused by diet, which is recognized to have an impact on human health (see the section “Gut Microbes, Nutrition, and Human Health” below). Given the positive health impacts illustrated by having a more rich and diverse gut microbiome, it is promising but not conclusive that contact with soil and its microbiome could be beneficial for animal health.
Supporting these findings, a study of baboons collected genetic material on 14 populations in southwest Kenya and examined 13 variables in each population’s environment (e.g., soil traits, vegetation) to understand the factors that supported gut microbiome membership and abundance across populations (Grieneisen et al. 2019). Neither host ancestry nor distance between populations were predictors of baboon gut microbial diversity. Instead, the effects from soil (namely geologic history and sodium content) were 15 times stronger than host population in predicting gut microbiome composition and diversity. It is possible that the baboons’ gut microbiomes
are being colonized through geophagy via consumption of soil microbes with their food. Regardless of the route, the study lends support to soils and their biotic content playing a structuring role in mammalian gut microbiomes. However, the exact mechanism of how soil and the environment more broadly shape the human gut microbiome, and particularly how healthy and degraded soils (and their microbiomes) could differentially affect the gut microbiome, needs further evaluation (Blum et al. 2019; Banerjee and van der Heijden 2023). While poorly resolved today, the relationship between soil health, soil microbiome, gut microbiome, and human health may have relevance for preventive medicine (Blum et al. 2019).
Gut Microbes, Nutrition, and Human Health
The largest concentration of microbes in the human body can be found in the large intestine, with roughly 1011 microbes per gram of intestinal content (Sender et al. 2016). The gut microbiome is the most widely studied ecosystem in humans. This section focuses on diet as a major mediator of microbiome composition and human health. Primary aspects of nutrient processing by gut microbes involve extraction of calories and nutrients from ingested foods, and production of a vast array of metabolites that can be absorbed by the host with organismal-wide benefits far beyond the origin of absorption in the gut. Some examples of microbial products in the gut lumen include amino acids, short-chain fatty acids (SCFA), methylamines, and cometabolites that result from metabolism of human-derived compounds such as bile acids.
Dietary intake can influence gut microbiome composition both in the short and long terms. On the one hand, individual gut microbiomes respond differently to identical meals (Zeevi et al. 2015; Korem et al. 2017; Johnson et al. 2019), while commonalities in dominant taxonomic groups appear with similar dietary patterns, such as vegetarian or omnivore (Wu et al. 2011; Gorvitovskaia et al. 2016; Figure 7-5). Certain dietary components may have a high potential to shape the gut microbiome and human health. For instance, the Fiber and Fermented Foods (FeFiFo) study demonstrated that a diet rich in fermented foods substantially increased microbial richness and decreased inflammatory markers (Wastyk et al. 2021).
Notably, in the same study, the impact of a high-fiber diet depended on individuals’ baseline level of fiber consumption, suggesting that the metabolic capacity of the gut microbiota may hinge on its composition before significant dietary changes. The bacteria in fermented foods may have accounted for the benefits observed in the FeFiFo study, which exemplifies how, in addition to nutrients and other compounds, microbes in foods can influence the composition of the gut microbiota. Astonishingly, even a single apple contains approximately 100 million bacterial cells, and the apple’s microbial richness can be shaped by agricultural management practices (Wassermann et al. 2019). Recent research highlighted the role of fruits and vegetables as sources of human gut microbial seeding (Wicaksono et al. 2023), revealing that foods can be a key linkage between the environmental microbiome (including the soil microbiome) and the human microbiome. This research underscores the significance of agricultural practices and plant microbial diversity for human health.
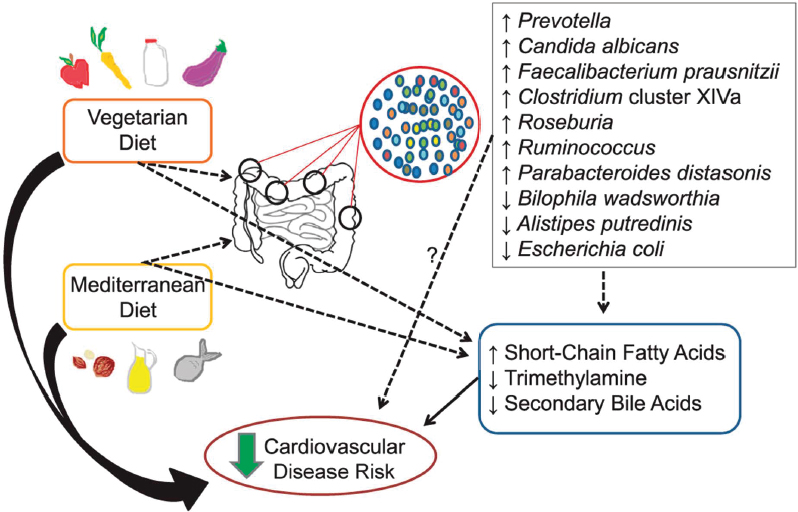
NOTE: The thick, solid-black arrows represent a large pool of evidence to support a pathway; narrow, solid-black arrows represent an intermediate amount of evidence to support a pathway; narrow, dotted black lines represent emerging evidence to support a pathway; and narrow, dotted black lines with a question mark represent other possible mechanistic pathways. Vegetarian and Mediterranean diets can alter the presence or absence of various bacteria and, in turn, also alter the gut metabolome. The direct effect of the gut microbial environment on cardiovascular disease risk is unknown. Vegetarian and Mediterranean diets can also affect the production of gut metabolites by serving as substrate for the resident bacteria.
SOURCE: Tindall et al. (2018). CCBY 4.0.
Dietary patterns may promote the expansion of taxonomic groups that have the metabolic capacity to process frequently consumed nutrients by (1) making these nutrients more bioavailable, (2) extracting calories from frequently consumed macronutrients, and (3) metabolizing nutrients into compounds with either health-promoting or disease-inducing properties. The latter pathway has been covered in previous sections of this chapter, so here the focus is on the influence of dietary patterns on microbiota composition and the role of gut microbes in human energy metabolism.
The involvement of gut microbes in energy metabolism was first observed in animal studies where germ-free rodents gained less weight than their conventional counterparts when consuming the same caloric load. It was later shown that the obese phenotype
could be transferred between mice through the microbiome (Turnbaugh et al. 2006; Bäckhed et al. 2007). The same phenomenon was observed when transferring fecal microbial samples from humans with obesity to germ-free mice in a diet-dependent manner (Ridaura et al. 2013). Animal studies have shown that energy metabolism, and in particular glucose and lipid homeostasis, are affected by the gut microbiome (Bäckhed et al. 2004; Caesar et al. 2015). Further, microbes and their metabolites can regulate hunger and satiety signaling as part of the gut-brain axis (Fetissov 2017).
Understanding the mechanisms underlying the regulation of energy metabolism is important in the context of both under- and over-nutrition, which are responsible for the greatest disease burdens around the world. Evidence thus far shows that the potential primary pathways for caloric extraction from macronutrients involve the production of metabolites from nutrient precursors by gut microbes. It has been suggested that the liberation of molecules such as proteins from the constant turnover of the bacterial biomass in the gut lumen contributes to energy extraction (Fetissov 2017). In rodents, modification of dietary fatty acids by gut microbes has been shown to generate lipid intermediates that may modify host lipid profiles (Kishino et al. 2013). Most notably, fiber fermentation yields SCFA such as butyrate, which serves as an energy source for colonocytes (Canfora et al. 2015; T. Chen et al. 2017). These microbially derived SCFA can modulate metabolic and anti-inflammatory pathways in the human host (Canfora et al. 2015; Nogal et al. 2021), but these mechanisms are not yet fully understood. The connections between fiber, the gut microbiome, and human health have been extensively reviewed elsewhere (Martens 2016; Holscher 2017; Sawicki et al. 2017).
Americans are far from aligning their diets with dietary guidelines and, in particular, from reaching daily dietary fiber recommendations (King et al. 2012). Intakes are especially low among low-income households and marginalized groups (McAnulty et al. 2017). Diets low in fiber, as seen, for example, in populations migrating to the United States, have been associated with low richness and diversity of gut microbiomes (Deehan and Walter 2016). Dietary patterns rich in fiber have been connected to multiple gastrointestinal and cardiometabolic health benefits (Anderson et al. 2009; Sawicki et al. 2017; McKeown et al. 2020). Strategies to promote fiber consumption are therefore urgently needed to promote human health.
CONCLUSIONS
The evidence strongly suggests that microorganisms create a link between the health of soils and the health of humans, but the processes by which microbiomes are established and influenced across these systems are still unexplored. The similarities and dissimilarities between soil and human microbiome composition and function need to be studied in detail while considering the environmental conditions and other contextual factors that may account for the lack of research reproducibility thus far. The committee proposes that attention be paid to the following areas.
Robust Sampling
Microbiome functional or compositional information could serve as early indicators of health. However, all microbiome indicators from biomass to gene expression traits lack universal standards, as these metrics are often context specific or relative to other samples and do not have universal cut-offs for what constitutes health responses. Moreover, it is uncertain how microbiome-derived indicators maintain their signals or diagnostic capabilities over time and changing external landscapes. Fortunately, microbiome sampling is becoming more cost-effective and efficiency in data processing is improving, enabling researchers to address the microbiome heterogeneity across relevant experimentally defined spatiotemporal scales, which offers a better understanding of the variation in microbiomes in both untreated and response to different treatments and health gradients. This knowledge combined with statistical approaches and a priori data exploration (both in sequencing depth and numbers of samples) can support funding for microbiome experiments designed with appropriate rigor for indicator discovery (Calgaro et al. 2020). These higher-dimensionality sampled microbiome data, combined with better reuse of existing data across studies, are needed to build more robust artificial learning models to discover microbiome indicators of health, uncovering local drivers and microbiome-enabled responsive indicators across axes of variation.
Recommendation 7-1: Researchers must incorporate sufficient rigor in the sampling design to capture the spatial and temporal heterogeneity of the microbiome to reveal responsive indicators of health.
Recommendation 7-2: Researchers should enhance universal methodologies (sampling, documentation) for microbiome analysis across different sample materials.
Data Tools and Data Management
It is posited that the next frontier in microbiome science lies in understanding the phenotype of a microbiome, the product of the combined genetic potential of the microbiome and available resources (Jansson and Hofmockel 2018). In microbiome science, linkages between phenotype and genotype are hindered by an inability to obtain most microorganisms in pure culture. Culture-independent multi-omic sequencing technologies, like those outlined in Figure 7-3, have the capacity to infer phenotypic features that would be otherwise difficult or time-consuming to assess experimentally. Inferring phenotype is considered one of the holy grails of biological research, as this knowledge can enhance predictive outcomes and provide targeted biotechnological applications in soil and human health realms. In fact, the integration of large-scale datasets with systematic meta-analysis has revolutionized biology, particularly in areas like translational research (Winkler et al. 2014). The ability to access and share well-annotated data collections allows for more robust genome-wide association studies and the application of
machine-learning techniques. These advancements underscore the importance of open data and standardized methods. Here, the collation of dozens of studies equates to the analysis of hundreds of thousands of experimental assays without lifting a pipette. Now, microbiome research is poised to leverage data sharing and reproduction for similar translational value to phenotypic exploration (Huttenhower et al. 2023).
To this end, the genomic repertoire of soil and human communities is ever more tractable today, spurred by federally enabled efforts like the HMP and the Genomic Encyclopedia of Bacteria and Archaea project, but also by research collectives like the Earth Microbiome Project and independently generated genomic compendiums (Almeida et al. 2021) that each generated hundreds of thousands of genomes from diverse sources. With the establishment of these massive sequencing catalogs, it is evident that a large portion of the species and functional diversity within human and soil microbiomes remain uncharacterized (Almeida et al. 2021). Thus, research efforts to experimentally characterize and computationally improve annotation of genomic content are urgently needed for accurate phenotypic extrapolations. Likewise, data management practices guided by FAIR principles are conduits for data reuse and mining, enabling further knowledge discovery and innovation. Incentivization by funding agencies and scholarly publishers for additional data that facilitates reuse—such as on environmental or land use properties for soil sequences or population characteristics for human sequences—will extend the collective value of these data collections beyond journal pages. As was true in 2007 when the first metagenomic report from the National Academies was published (NRC 2007), funding sources that create and enable appropriate data management resources are needed.
The committee recognizes data management in microbiology presents a multifaceted challenge, particularly when it comes to the collection and maintenance of metadata. While funding for research endeavors is crucial, it is often overlooked that explicit allocation for metadata collection is essential for comprehensive data management. However, the process of gathering robust metadata is far from simple. Balancing the need for robust metadata against the practical limitations of available resources is a persistent issue. Without adequate support, the quality and depth of metadata suffer, undermining the integrity and utility of research findings. It is a dilemma that requires careful consideration and realistic solutions, as simply expecting exhaustive metadata without appropriate funding is unrealistic and unsustainable within existing grant structures. With increasing awareness in these directions, microbiome-based data sciences and applications can transition from a discipline of description to one that delivers more novel and surprising technologies.
Recommendation 7-3: Federal funders should require resources to ensure metadata as well as data on environmental and ecosystem properties or population characteristics be included, properly stored, and reusable, as accessible data are necessary but not enough.
Diagnostics
New analytical and conceptual approaches will likely be developed that capture microbiome-based systems characteristic of health in both soils and humans. These advances will operationalize both the monitoring of health but also further understanding of how microbiomes influence the stability and functioning of ecosystems and how these underlying properties scale to provide qualities of health or wellness. More efficient measurements of microbiome content with chemical constituents, which could be enhanced by precision or digital agricultural infrastructures and more thorough assessment of human ecosystems along time and special dimensions, are avenues that will be leveraged for quantifying microbiome diagnostics of health. A goal would be to move from correlation to predictive outcomes.
Recommendation 7-4: Funding agencies should support discovery of scalable diagnostics, with the goal that affordable, rapid assays will be developed for use on soil microbiomes in the field and with diverse human populations.
Recommendation 7-5: Funding agencies should support research designed to investigate causal relationships in soil and human microbiomes, toward the development of microbial therapeutics.
Interdisciplinary Research
Microbial ecosystems are an important link in the continuum of soil health and human health. There are a variety of direct (e.g., exposure to dust and contaminants) and indirect (e.g., quality of crops and bioavailability of nutrients) pathways from soil to human health that are deeply affected by microbial composition and function. However, the evidence thus far is mostly correlational and needs validation and replication. With the ever-increasing challenges posed by human activities and climate change, it is imperative to further unveil the mechanistic links underlying microbiota composition and function, soil health, and human health. Furthermore, parallel processes whereby microbiome function influences soil, plant, and animal health should be leveraged in the development of strategies to mitigate emerging challenges to the health of these systems. As was noted in the 2007 National Academies report on metagenomics (NRC 2007), overcoming such challenges will require a systems approach across and within disciplines, supported by multiple funding agencies, to explore the existence of the microbiome continuum spanning soils to human health.
Recommendation 7-6: Funding agencies should support microbiome research within disciplines (e.g., community ecology, soil ecology, and soil biogeochemistry or microbiology and medicine) to integrate methodologies to bring together composition and functional assessments of microbiomes.
Recommendation 7-7: Funding agencies should support microbiome research among disciplines (e.g., agronomy, plant science, soil ecology, microbiology, immunology, human nutrition, medicine, engineering) to explore the connectivity of the microbiome across systems (e.g., soil, plants, and humans).
REFERENCES
Aderemi, Adewale Victor, Ademola Olabode Ayeleso, Oluboade Oluokun Oyedapo, and Emmanuel Mukwevho. 2021. “Metabolomics: A Scoping Review of Its Role as a Tool for Disease Biomarker Discovery in Selected Non-Communicable Diseases.” Metabolites 11 (7): 418. https://doi.org/10.3390/metabo11070418.
Aguiar-Pulido, Vanessa, Wenrui Huang, Victoria Suarez-Ulloa, Trevor Cickovski, Kalai Mathee, and Giri Narasimhan. 2016. “Metagenomics, Metatranscriptomics, and Metabolomics Approaches for Microbiome Analysis: Supplementary Issue: Bioinformatics Methods and Applications for Big Metagenomics Data.” Evolutionary Bioinformatics 12s1: EBO.S36436. https://doi.org/10.4137/ebo.S36436.
Akdis, Cezmi A. 2021. “Does the Epithelial Barrier Hypothesis Explain the Increase in Allergy, Autoimmunity and Other Chronic Conditions?” Nature Reviews Immunology 21 (11): 739−751. https://doi.org/10.1038/s41577-021-00538-7.
Almeida, Alexandre, Stephen Nayfach, Miguel Boland, Francesco Strozzi, Martin Beracochea, Zhou Jason Shi, Katherine S. Pollard, Ekaterina Sakharova, Donovan H. Parks, Philip Hugenholtz, Nicola Segata, Nikos C. Kyrpides, and Robert D. Finn. 2021. “A Unified Catalog of 204,938 Reference Genomes from the Human Gut Microbiome.” Nature Biotechnology 39 (1): 105−114. https://doi.org/10.1038/s41587-020-0603-3.
Altheide, S. Travis 2020. “Biochemical and Culture-Based Approaches to Identification in the Diagnostic Microbiology Laboratory.” American Society for Clinical Laboratory Science: ascls.119.001875. https://doi.org/10.29074/ascls.119.001875.
Ames, Nancy J., Alexandra Ranucci, Brad Moriyama, and Gwenyth R. Wallen. 2017. “The Human Microbiome and Understanding the 16S rRNA Gene in Translational Nursing Science.” Nursing Research 66 (2). https://doi.org/10.1097/nnr.0000000000000212.
Anderson, James W., Pat Baird, Richard H. Davis, Jr, Stefanie Ferreri, Mary Knudtson, Ashraf Koraym, Valerie Waters, and Christine L. Williams. 2009. “Health Benefits of Dietary Fiber.” Nutrition Reviews 67 (4): 188−205. https://doi.org/10.1111/j.1753-4887.2009.00189.x.
Asnicar, Francesco, Andrew Maltez Thomas, Andrea Passerini, Levi Waldron, and Nicola Segata. 2024. “Machine Learning for Microbiologists.” Nature Reviews Microbiology 22 (4): 191−205. https://doi.org/10.1038/s41579-023-00984-1.
Bach, Jean-François. 2018. “The Hygiene Hypothesis in Autoimmunity: The Role of Pathogens and Commensals.” Nature Reviews Immunology 18 (2): 105−120. https://doi.org/10.1038/nri.2017.111.
Bäckhed, Fredrik, Hao Ding, Ting Wang, Lora V. Hooper, Gou Young Koh, Andras Nagy, Clay F. Semenkovich, and Jeffrey I. Gordon. 2004. “The Gut Microbiota as an Environmental Factor That Regulates Fat Storage.” Proceedings of the National Academy of Sciences 101 (44): 15718−15723. https://doi.org/10.1073/pnas.0407076101.
Bäckhed, Fredrik, Jill K. Manchester, Clay F. Semenkovich, and Jeffrey I. Gordon. 2007. “Mechanisms Underlying the Resistance to Diet-induced Obesity in Germ-Free Mice.” Proceedings of the National Academy of Sciences 104 (3): 979−984. https://doi.org/10.1073/pnas.0605374104.
Bahadur, Ali, Asfa Batool, Fahad Nasir, Shengjin Jiang, Qin Mingsen, Qi Zhang, Jianbin Pan, Yongjun Liu, and Huyuan Feng. 2019. “Mechanistic Insights into Arbuscular Mycorrhizal Fungi-Mediated Drought Stress Tolerance in Plants.” International Journal of Molecular Sciences 20 (17): 4199. https://www.mdpi.com/1422-0067/20/17/4199.
Banerjee, Joyita, Neetu Mishra, and Yogita Dhas. 2015. “Metagenomics: A New Horizon in Cancer Research.” Meta Gene 5: 84−89. https://doi.org/10.1016/j.mgene.2015.05.005.
Banerjee, Samiran, and Marcel G. A. van der Heijden. 2023. “Soil Microbiomes and One Health.” Nature Reviews Microbiology 21 (1): 6−20. https://doi.org/10.1038/s41579-022-00779-w.
Banerjee, Samiran, Florian Walder, Lucie Büchi, Marcel Meyer, Alain Y. Held, Andreas Gattinger, Thomas Keller, Raphael Charles, and Marcel G. A. van der Heijden. 2019. “Agricultural Intensification Reduces Microbial Network Complexity and the Abundance of Keystone Taxa in Roots.” The ISME Journal 13 (7): 1722−1736. https://doi.org/10.1038/s41396-019-0383-2.
Barabási, Albert-László, Natali Gulbahce, and Joseph Loscalzo. 2011. “Network Medicine: A Network-Based Approach to Human Disease.” Nature Reviews Genetics 12 (1): 56−68. https://doi.org/10.1038/nrg2918.
Berg, Gabriele, Daria Rybakova, Doreen Fischer, Tomislav Cernava, Marie-Christine Champomier Vergès, Trevor Charles, Xiaoyulong Chen, Luca Cocolin, Kellye Eversole, Gema Herrero Corral, Maria Kazou, Linda Kinkel, Lene Lange, Nelson Lima, Alexander Loy, James A. Macklin, Emmanuelle Maguin, Tim Mauchline, Ryan McClure, Birgit Mitter, Matthew Ryan, Inga Sarand, Hauke Smidt, Bettina Schelkle, Hugo Roume, G. Seghal Kiran, Joseph Selvin, Rafael Soares Correa de Souza, Leo van Overbeek, Brajesh K. Singh, Michael Wagner, Aaron Walsh, Angela Sessitsch, and Michael Schloter. 2020. “Microbiome Definition Re-visited: Old Concepts and New Challenges.” Microbiome 8 (1): 103. https://doi.org/10.1186/s40168-020-00875-0.
Bertola, Marta, Andrea Ferrarini, and Giovanna Visioli. 2021. “Improvement of Soil Microbial Diversity through Sustainable Agricultural Practices and Its Evaluation by -Omics Approaches: A Perspective for the Environment, Food Quality and Human Safety.” Microorganisms 9 (7): 1400. https://doi.org/10.1016/j.agee.2011.01.017.
Bhagchandani, Tannu, Nikita, Anjali Verma, and Ravi Tandon. 2023. “Exploring the Human Virome: Composition, Dynamics, and Implications for Health and Disease.” Current Microbiology 81 (1): 16. https://doi.org/10.1007/s00284-023-03537-0.
Blum, Winfried E. H., Sophie Zechmeister-Boltenstern, and Katharina M. Keiblinger. 2019. “Does Soil Contribute to the Human Gut Microbiome?” Microorganisms 7 (9): 287. https://doi.org/10.3390%2Fmicroorganisms7090287.
Bollmann-Giolai, Anita, Jacob G. Malone, and Sanu Arora. 2022. “Diversity, Detection and Exploitation: Linking Soil Fungi and Plant Disease.” Current Opinion in Microbiology 70: 102199. https://doi.org/10.1016/j.mib.2022.102199.
Borton, Mikayla A., Michael Shaffer, David W. Hoyt, Ruisheng Jiang, Jared B. Ellenbogen, Samuel Purvine, Carrie D. Nicora, Elizabeth K. Eder, Allison R. Wong, A. George Smulian, Mary S. Lipton, Joseph A. Krzycki, and Kelly C. Wrighton. 2023. “Targeted Curation of the Gut Microbial Gene Content Modulating Human Cardiovascular Disease.” mBio 14 (5): e01511-23. https://doi.org/10.1128/mbio.01511-23.
Brooks, J. Paul, Gregory A. Buck, Guanhua Chen, Liyang Diao, David J. Edwards, Jennifer M. Fettweis, Snehalata Huzurbazar, Alexander Rakitin, Glen A. Satten, Ekaterina Smirnova, Zeev Waks, Michelle L. Wright, Chen Yanover, and Yi-Hui Zhou. 2017. “Changes in Vaginal Community State Types Reflect Major Shifts in the Microbiome.” Microbial Ecology in Health and Disease 28 (1): 1303265. https://doi.org/10.1080/16512235.2017.1303265.
Caesar, Robert, Valentina Tremaroli, Petia Kovatcheva-Datchary, Patrice D. Cani, and Fredrik Bäckhed. 2015. “Crosstalk Between Gut Microbiota and Dietary Lipids Aggravates WAT Inflammation through TLR Signaling.” Cell Metabolism 22 (4): 658−668. https://doi.org/10.1016/j.cmet.2015.07.026.
Calderone, Alberto, Luana Licata, and Gianni Cesareni. 2015. “VirusMentha: A New Resource for Virus-Host Protein Interactions.” Nucleic Acids Research 43 (D1): D588−D592. https://doi.org/10.1093/nar/gku830.
Calgaro, Matteo, Chiara Romualdi, Levi Waldron, Davide Risso, and Nicola Vitulo. 2020. “Assessment of Statistical Methods from Single Cell, Bulk RNA-seq, and Metagenomics Applied to Microbiome Data.” Genome Biology 21 (1): 191. https://doi.org/10.1186/s13059020-02104-1.
Canfora, Emanuel E., Johan W. Jocken, and Ellen E. Blaak. 2015. “Short-Chain Fatty Acids in Control of Body Weight and Insulin Sensitivity.” Nature Reviews Endocrinology 11 (10): 577−591. https://doi.org/10.1038/nrendo.2015.128.
Carini, Paul, Patrick J. Marsden, Jonathan W. Leff, Emily E. Morgan, Michael S. Strickland, and Noah Fierer. 2016. “Relic DNA Is Abundant in Soil and Obscures Estimates of Soil Microbial Diversity.” Nature Microbiology 2 (3): 16242. https://doi.org/10.1038/nmicrobiol.2016.242.
Chagas, César da Silva, Waldir de Carvalho Junior, Silvio Barge Bhering, and Braz Calderano Filho. 2016. “Spatial Prediction of Soil Surface Texture in a Semiarid Region Using Random Forest and Multiple Linear Regressions.” CATENA 139: 232−240. https://doi.org/10.1016/j.catena.2016.01.001.
Chen, Fang, Shenghui Li, Ruochun Guo, Fanghua Song, Yue Zhang, Xifan Wang, Xiaokui Huo, Qingbo Lv, Hayan Ullah, Guangyang Wang, Yufang Ma, Qiulong Yan, and Xiaochi Ma. 2023. “Meta-analysis of Fecal Viromes Demonstrates High Diagnostic Potential of the Gut Viral Signatures for Colorectal Cancer and Adenoma Risk Assessment.” Journal of Advanced Research 49: 103−114. https://doi.org/10.1016/j.jare.2022.09.012.
Chen, Lianmin, Valerie Collij, Martin Jaeger, Inge C. L. van den Munckhof, Arnau Vich Vila, Alexander Kurilshikov, Ranko Gacesa, Trishla Sinha, Marije Oosting, Leo A. B. Joosten, Joost H. W. Rutten, Niels P. Riksen, Ramnik J. Xavier, Folkert Kuipers, Cisca Wijmenga, Alexandra Zhernakova, Mihai G. Netea, Rinse K. Weersma, and Jingyuan Fu. 2020. “Gut Microbial Co-abundance Networks Show Specificity in Inflammatory Bowel Disease and Obesity.” Nature Communications 11 (1): 4018. https://doi.org/10.1038/s41467-020-17840-y.
Chen, Tingting, Wenmin Long, Chenhong Zhang, Shuang Liu, Liping Zhao, and Bruce R. Hamaker. 2017. “Fiber-Utilizing Capacity Varies in Prevotella- versus Bacteroides-Dominated Gut Microbiota.” Scientific Reports 7 (1): 2594. https://doi.org/10.1038/s41598-017-02995-4.
Chepsergon, Jane, and Lucy N. Moleleki. 2023. “Rhizosphere Bacterial Interactions and Impact on Plant Health.” Current Opinion in Microbiology 73: 102297. https://doi.org/10.1016/j.mib.2023.102297.
Chinda, Daisuke, Toshihiko Takada, Tatsuya Mikami, Kensuke Shimizu, Kosuke Oana, Tetsu Arai, Kazuki Akitaya, Hirotake Sakuraba, Miyuki Katto, Yusuke Nagara, Hiroshi Makino, Daichi Fujii, Kenji Oishi, and Shinsaku Fukuda. 2022. “Spatial Distribution of Live Gut Microbiota and Bile Acid Metabolism in Various Parts of Human Large Intestine.” Scientific Reports 12, 3593. https://doi.org/10.1038/s41598-022-07594-6
Cho, Ilseung, and Martin J. Blaser. 2012. “The Human Microbiome: At the Interface of Health and Disease.” Nature Reviews Genetics 13 (4): 260−270. https://doi.org/10.1038/nrg3182.
Crits-Christoph, Alexander, Spencer Diamond, Cristina N. Butterfield, Brian C. Thomas, and Jillian F. Banfield. 2018. “Novel Soil Bacteria Possess Diverse Genes for Secondary Metabolite Biosynthesis.” Nature 558 (7710): 440−444. https://doi.org/10.1038/s41586-018-0207-y.
d’Humières, Camille, Maud Salmona, Sarah Dellière, Stefano Leo, Christophe Rodriguez, Cécile Angebault, Alexandre Alanio, Slim Fourati, Vladimir Lazarevic, Paul-Louis Woerther, Jacques Schrenzel, and Etienne Ruppé. 2021. “The Potential Role of Clinical Metagenomics in Infectious Diseases: Therapeutic Perspectives.” Drugs 81, 1453–1466. https://doi.org/10.1007/s40265-021-01572-4.
Dagenais, Amy, Carlos Villalba-Guerrero, and Martin Olivier. 2023. “Trained Immunity: A ‘New’ Weapon in the Fight Against Infectious Diseases.” Frontiers in Immunology 14. https://doi.org/10.3389/fimmu.2023.1147476.
Das Purkayastha, Sumi, Mrinal K. Bhattacharya, Himanshu K. Prasad, and Surajit De Mandal. 2020. “Chapter 17 - Diversity and the Antimicrobial Activity of Vaginal Lactobacilli: Current Status and Future Prospective.” In Recent Advancements in Microbial Diversity, edited by Surajit De Mandal and Pankaj Bhatt, 397−422. Academic Press.
Deehan, Edward C., and Jens Walter. 2016. “The Fiber Gap and the Disappearing Gut Microbiome: Implications for Human Nutrition.” Trends in Endocrinology & Metabolism 27 (5): 239−242. https://doi.org/10.1016/j.tem.2016.03.001.
Delgado-Baquerizo, Manuel, Jasmine Grinyer, Peter B. Reich, and Brajesh K. Singh. 2016a. “Relative Importance of Soil Properties and Microbial Community for Soil Functionality: Insights from a Microbial Swap Experiment.” Functional Ecology 30 (11): 1862−1873. https://doi.org/10.1111/1365-2435.12674.
Delgado-Baquerizo, Manuel, Fernando T. Maestre, Peter B. Reich, Thomas C. Jeffries, Juan J. Gaitan, Daniel Encinar, Miguel Berdugo, Colin D. Campbell, and Brajesh K. Singh. 2016b. “Microbial Diversity Drives Multifunctionality in Terrestrial Ecosystems.” Nature Communications 7 (1): 10541. https://doi.org/10.1038/ncomms10541.
DeLuca, Thomas H., Melissa R. A. Pingree, and Si Gao. 2019. “Chapter 16 - Assessing Soil Biological Health in Forest Soils.” In Global Change and Forest Soils, edited by Matt Busse, Christian P. Giardina, Dave M. Morris, and Debbie S. Page-Dumroese, 397−426. Amsterdam: Elsevier.
Di Rienzi, Sara C., Itai Sharon, Kelly C. Wrighton, Omry Koren, Laura A. Hug, Brian C. Thomas, Julia K. Goodrich, Jordana T. Bell, Timothy D. Spector, Jillian F. Banfield, and Ruth E. Ley. 2013. “The Human Gut and Groundwater Harbor Non-photosynthetic Bacteria Belonging to a New Candidate Phylum Sibling to Cyanobacteria.” Elife 2: e01102. https://doi.org/10.7554/eLife.01102.
Domazet-Lošo, Tomislav, and Diethard Tautz. 2008. “An Ancient Evolutionary Origin of Genes Associated with Human Genetic Diseases.” Molecular Biology and Evolution 25 (12): 2699−2707. https://doi.org/10.1093/molbev/msn214.
Donovan, Paul D., Gabriel Gonzalez, Desmond G. Higgins, Geraldine Butler, and Kimihito Ito. 2018. “Identification of Fungi in Shotgun Metagenomics Datasets.” PLOS ONE 13 (2): e0192898. https://doi.org/10.1371/journal.pone.0192898.
Douglas, Gavin M., Vincent J. Maffei, Jesse R. Zaneveld, Svetlana N. Yurgel, James R. Brown, Christopher M. Taylor, Curtis Huttenhower, and Morgan G. I. Langille. 2020. “PICRUSt2 for Prediction of Metagenome Functions.” Nature Biotechnology 38 (6): 685−688. https://doi.org/10.1038/s41587-020-0548-6.
Dundore-Arias, J. P., M. Michalska-Smith, M. Millican, and L. L. Kinkel. 2023. “More Than the Sum of Its Parts: Unlocking the Power of Network Structure for Understanding Organization and Function in Microbiomes.” Annual Review of Phytopathology 61 (1): 403−423. https://doi.org/10.1146/annurev-phyto-021021-041457.
Duque Zapata, Juan Diego, Jaime Eduardo Muñoz Florez, and Diana Lopez Alvarez. 2023. “Metagenomics Approaches to Understanding Soil Health in Environmental Research - A Review.” Soil Science Annual 74 (1): 1−11. https://doi.org/10.37501/soilsa/163080.
Durán, Paloma, Thorsten Thiergart, Ruben Garrido-Oter, Matthew Agler, Eric Kemen, Paul Schulze-Lefert, and Stéphane Hacquard. 2018. “Microbial Interkingdom Interactions in Roots Promote Arabidopsis Survival.” Cell 175 (4): 973−983.e14. https://doi.org/10.1016/j.cell.2018.10.020.
Eisenstein, Michael. 2020. “The Hunt for a Healthy Microbiome.” Nature 577 (7792): S6−S8. https://doi.org/10.1038/d41586-020-00193-3.
Ellenbogen, Jared B., Mikayla A. Borton, Bridget B. McGivern, Dylan R. Cronin, David W. Hoyt, Viviana Freire-Zapata, Carmody K. McCalley, Ruth K. Varner, Patrick M. Crill, Richard A. Wehr, Jeffrey P. Chanton, Ben J. Woodcroft, Malak M. Tfaily, Gene W. Tyson, Virginia I. Rich, and Kelly C. Wrighton. 2024. “Methylotrophy in the Mire: Direct and Indirect Routes for Methane Production in Thawing Permafrost.” mSystems 9 (1): e00698-23. https://doi.org/10.1128/msystems.00698-23.
Emerson, Joanne B., Simon Roux, Jennifer R. Brum, Benjamin Bolduc, Ben J. Woodcroft, Ho Bin Jang, Caitlin M. Singleton, Lindsey M. Solden, Adrian E. Naas, Joel A. Boyd, Suzanne B. Hodgkins, Rachel M. Wilson, Gareth Trubl, Changsheng Li, Steve Frolking, Phillip B. Pope, Kelly C. Wrighton, Patrick M. Crill, Jeffrey P. Chanton, Scott R. Saleska, Gene W. Tyson, Virginia I. Rich, and Matthew B. Sullivan. 2018. “Host-Linked Soil Viral Ecology Along a Permafrost Thaw Gradient.” Nature Microbiology 3 (8): 870−880. https://doi.org/10.1038/s41564-018-0190-y.
Falony, Gwen, Marie Joossens, Sara Vieira-Silva, Jun Wang, Youssef Darzi, Karoline Faust, Alexander Kurilshikov, Marc Jan Bonder, Mireia Valles-Colomer, Doris Vandeputte, Raul Tito Tadeo, Samuel Chaffron, Leen Rymenans, Chloë Verspecht, Lise Sutter, Gipsi Lima-Mendez, Kevin D’hoe, Karl Jonckheere, Daniel Homola, and Jeroen Raes. 2016. “Population-Level Analysis of Gut Microbiome Variation.” Science 352: 560−564. https://doi.org/10.1126/science.aad3503.
Fan, Yong, and Oluf Pedersen. 2021. “Gut Microbiota in Human Metabolic Health and Disease.” Nature Reviews Microbiology 19 (1): 55−71. https://doi.org/10.1038/s41579-020-0433-9.
FAO, UNEP, WHO, and WOAH (Food and Agriculture Organization of the United Nations, United Nations Environment Programme, World Health Organization, and World Organisation for Animal Health). 2022. One Health Joint Plan of Action (2022–2026). Working Together for the Health of Humans, Animals, Plants and the Environment. Rome. https://doi.org/10.4060/cc2289en.
Fassarella, Marina, Ellen E. Blaak, John Penders, Arjen Nauta, Hauke Smidt Hauke, and Erwin G. Zoetendal. 2021. “Gut Microbiome Stability and Resilience: Elucidating the Response to Perturbations in Order to Modulate Gut Health.” Gut 70 (3): 595. https://doi.org/10.1136/gutjnl-2020-321747.
Ferrocino, Ilario, Kalliopi Rantsiou, Ryan McClure, Tanja Kostic, Rafael Soares Correa de Souza, Lene Lange, Jamie FitzGerald, Aicha Kriaa, Paul Cotter, Emmanuelle Maguin, Bettina Schelkle, Michael Schloter, Gabriele Berg, Angela Sessitsch, Luca Cocolin, and The MicrobiomeSupport Consortium. 2023. “The Need for an Integrated Multi-OMICs Approach in Microbiome Science in the Food System.” Comprehensive Reviews in Food Science and Food Safety 22 (2): 1082−1103. https://doi.org/10.1111/1541-4337.13103.
Fetissov, Sergueï O. 2017. “Role of the Gut Microbiota in Host Appetite Control: Bacterial Growth to Animal Feeding Behaviour.” Nature Reviews Endocrinology 13 (1): 11−25. https://doi.org/10.1038/nrendo.2016.150.
Fierer, Noah, Stephen A. Wood, and Clifton P. Bueno de Mesquita. 2021. “How Microbes Can, and Cannot, Be Used to Assess Soil Health.” Soil Biology and Biochemistry 153: 108111. https://doi.org/10.1016/j.soilbio.2020.108111.
Fitzpatrick, Connor R., Julia Copeland, Pauline W. Wang, David S. Guttman, Peter M. Kotanen, and Marc T. J. Johnson. 2023. “Habitats Within the Plant Root Differ in Bacterial Network Topology and Taxonomic Assortativity.” Molecular Plant-Microbe Interactions® 36 (3): 165−175. https://doi.org/10.1094/mpmi-09-22-0188-r.
Frąc, Magdalena, Silja E. Hannula, Marta Bełka, and Małgorzata Jędryczka. 2018. “Fungal Biodiversity and Their Role in Soil Health.” Frontiers in Microbiology 9. https://doi.org/10.3389/fmicb.2018.00707.
France, Michael, Madeline Alizadeh, Sarah Brown, Bing Ma, and Jacques Ravel. 2022. “Towards a Deeper Understanding of the Vaginal Microbiota.” Nature Microbiology 7 (3): 367−378. https://doi.org/10.1038/s41564-022-01083-2.
Fujita, Hiroaki, Masayuki Ushio, Kenta Suzuki, Masato S. Abe, Masato Yamamichi, Koji Iwayama, Alberto Canarini, Ibuki Hayashi, Keitaro Fukushima, Shinji Fukuda, E. Toby Kiers, and Hirokazu Toju. 2023. “Alternative Stable States, Nonlinear Behavior, and Predictability of Microbiome Dynamics.” Microbiome 11 (1): 63. https://doi.org/10.1186/s40168-02301474-5.
Gacesa, R., A. Kurilshikov, A. Vich Vila, T. Sinha, M. A. Y. Klaassen, L. A. Bolte, S. Andreu-Sánchez, L. Chen, V. Collij, S. Hu, J. A. M. Dekens, V. C. Lenters, J. R. Björk, J. C. Swarte, M. A. Swertz, B. H. Jansen, J. Gelderloos-Arends, S. Jankipersadsing, M. Hofker, R. C. H. Vermeulen, S. Sanna, H. J. M. Harmsen, C. Wijmenga, J. Fu, A. Zhernakova, and R. K. Weersma. 2022. “Environmental Factors Shaping the Gut Microbiome in a Dutch Population.” Nature 604 (7907): 732−739. https://doi.org/10.1038/s41586-022-04567-7.
Gao, Yilin, Zifan Zhu, and Fengzhu Sun. 2022. “Increasing Prediction Performance of Colorectal Cancer Disease Status Using Random Forests Classification Based on Metagenomic Shotgun Sequencing Data.” Synthetic and Systems Biotechnology 7 (1): 574−585. https://doi.org/10.1016/j.synbio.2022.01.005.
Genin, Stéphane, and Timothy P. Denny. 2012. “Pathogenomics of the Ralstonia solanacearum Species Complex.” Annual Review of Phytopathology 50 (1): 67−89. https://doi.org/10.1146/annurev-phyto-081211-173000.
German, J. Bruce, Bruce D. Hammock, and Steven M. Watkins. 2005. “Metabolomics: Building on a Century of Biochemistry to Guide Human Health.” Metabolomics 1 (1): 3−9. https://doi.org/10.1007/s11306-005-1102-8.
Ghimire, Rajan, Vesh R. Thapa, Veronica Acosta-Martinez, Meagan Schipanski, Lindsey C. Slaughter, Steven J. Fonte, Manoj K. Shukla, Prakriti Bista, Sangamesh V. Angadi, Maysoon M. Mikha, Olufemi Adebayo, and Tess Noble Strohm. 2023. “Soil Health Assessment and Management Framework for Water-Limited Environments: Examples from the Great Plains of the USA.” Soil Systems 7 (1): 22. https://www.mdpi.com/2571-8789/7/1/22.
Gilbert, Jack A., Martin J. Blaser, J. Gregory Caporaso, Janet K. Jansson, Susan V. Lynch, and Rob Knight. 2018. “Current Understanding of the Human Microbiome.” Nature Medicine 24 (4): 392−400. https://doi.org/10.1038/nm.4517.
Gorvitovskaia, Anastassia, Susan P. Holmes, and Susan M. Huse. 2016. “Interpreting Prevotella and Bacteroides as Biomarkers of Diet and Lifestyle.” Microbiome 4 (1): 15. https://doi.org/10.1186/s40168-016-0160-7.
Gregory, Ann C., Matthew B. Sullivan, Leopoldo N. Segal, and Brian C. Keller. 2018. “Smoking Is Associated with Quantifiable Differences in the Human Lung DNA Virome and Metabolome.” Respiratory Research 19 (1): 174. https://doi.org/10.1186/s12931-018-0878-9.
Grieneisen, Laura E., Marie J. E. Charpentier, Susan C. Alberts, Ran Blekhman, Gideon Bradburd, Jenny Tung, and Elizabeth A. Archie. 2019. “Genes, Geology and Germs: Gut Microbiota Across a Primate Hybrid Zone Are Explained by Site Soil Properties, Not Host Species.” Proceedings of the Royal Society B: Biological Sciences 286 (1901): 20190431. https://doi.org/10.1098/rspb.2019.0431.
Gu, Yian, Samiran Banerjee, Francisco Dini-Andreote, Yangchun Xu, Qirong Shen, Alexandre Jousset, and Zhong Wei. 2022. “Small Changes in Rhizosphere Microbiome Composition Predict Disease Outcomes Earlier than Pathogen Density Variations.” The ISME Journal 16 (10): 2448−2456. https://doi.org/10.1038/s41396-022-01290-z.
Hajishengallis, George, Richard P. Darveau, and Michael A. Curtis. 2012. “The Keystone-Pathogen Hypothesis.” Nature Reviews Microbiology 10 (10): 717−725. https://doi.org/10.1038/nrmicro2873.
Halfvarson, Jonas, Colin J. Brislawn, Regina Lamendella, Yoshiki Vázquez-Baeza, William A. Walters, Lisa M. Bramer, Mauro D’Amato, Ferdinando Bonfiglio, Daniel McDonald, Antonio Gonzalez, Erin E. McClure, Mitchell F. Dunklebarger, Rob Knight, and Janet K. Jansson. 2017. “Dynamics of the Human Gut Microbiome in Inflammatory Bowel Disease.” Nature Microbiology 2 (5): 17004. https://doi.org/10.1038/nmicrobiol.2017.4.
Hanski, Ilkka, Leena von Hertzen, Nanna Fyhrquist, Kaisa Koskinen, Kaisa Torppa, Tiina Laatikainen, Piia Karisola, Petri Auvinen, Lars Paulin, Mika J. Mäkelä, Erkki Vartiainen, Timo U. Kosunen, Harri Alenius, and Tari Haahtela. 2012. “Environmental Biodiversity, Human Microbiota, and Allergy Are Interrelated.” Proceedings of the National Academy of Sciences 109 (21): 8334−8339. https://doi.org/10.1073/pnas.1205624109.
Hariharan, Ganeshamoorthy, and Kandeeparoopan Prasannath. 2021. “Recent Advances in Molecular Diagnostics of Fungal Plant Pathogens: A Mini Review.” Frontiers in Cellular and Infection Microbiology 10. https://doi.org/10.3389/fcimb.2020.600234.
Harvey, Eric, Isabelle Gounand, Colette L. Ward, and Florian Altermatt. 2017. “Bridging Ecology and Conservation: From Ecological Networks to Ecosystem Function.” Journal of Applied Ecology 54 (2): 371−379. https://doi.org/10.1111/1365-2664.12769.
Hawkins, Heidi-Jayne, Rachael I. M. Cargill, Michael E. Van Nuland, Stephen C. Hagen, Katie J. Field, Merlin Sheldrake, Nadejda A. Soudzilovskaia, and E. Toby Kiers. 2023. “Mycorrhizal Mycelium as a Global Carbon Pool.” Current Biology 33 (11): R560−R573. https://doi.org/10.1016/j.cub.2023.02.027.
Hernández Medina, Ricardo, Svetlana Kutuzova, Knud Nor Nielsen, Joachim Johansen, Lars Hestbjerg Hansen, Mads Nielsen, and Simon Rasmussen. 2022. “Machine Learning and Deep Learning Applications in Microbiome Research.” ISME Communications 2 (1): 98. https://doi.org/10.1038/s43705-022-00182-9.
Heung, Brandon, Chuck E. Bulmer, and Margaret G. Schmidt. 2014. “Predictive Soil Parent Material Mapping at a Regional-Scale: A Random Forest Approach.” Geoderma 214−215: 141−154. https://doi.org/10.1016/j.geoderma.2013.09.016.
HMP (The Integrative HMP Research Network Consortium). 2019. “The Integrative Human Microbiome Project.” Nature 569 (7758): 641−648. https://doi.org/10.1038/s41586-0191238-8.
Hodinka, Richard L., and Laurent Kaiser. 2013. “Point-Counterpoint: Is the Era of Viral Culture Over in the Clinical Microbiology Laboratory?” Journal of Clinical Microbiology 51 (1): 2−8. https://doi.org/10.1128/jcm.02593-12.
Holscher, Hannah D. 2017. “Dietary Fiber and Prebiotics and the Gastrointestinal Microbiota.” Gut Microbes 8 (2): 172−184. https://doi.org/10.1080/19490976.2017.1290756.
Hou, Kaijian, Zhuo-Xun Wu, Xuan-Yu Chen, Jing-Quan Wang, Dongya Zhang, Chuanxing Xiao, Dan Zhu, Jagadish B. Koya, Liuya Wei, Jilin Li, and Zhe-Sheng Chen. 2022. “Microbiota in Health and Diseases.” Signal Transduction and Targeted Therapy 7 (1): 135. https://doi.org/10.1038/s41392-022-00974-4.
Hungate, Bruce A., Rebecca L. Mau, Egbert Schwartz, J. Gregory Caporaso, Paul Dijkstra, Natasja van Gestel, Benjamin J. Koch, Cindy M. Liu, Theresa A. McHugh, Jane C. Marks, Ember M. Morrissey, and Lance B. Price. 2015. “Quantitative Microbial Ecology through Stable Isotope Probing.” Applied and Environmental Microbiology 81 (21): 7570−7581. https://doi.org/10.1128/AEM.02280-15.
Huttenhower, Curtis, Robert D. Finn, and Alice Carolyn McHardy. 2023. “Challenges and Opportunities in Sharing Microbiome Data and Analyses.” Nature Microbiology 8 (11): 1960−1970. https://doi.org/10.1038/s41564-023-01484-x.
Issa Isaac, Ngom, Decloquement Philippe, Armstrong Nicholas, Didier Raoult, and Chabrière Eric. 2019. “Metaproteomics of the Human Gut Microbiota: Challenges and Contributions to Other OMICS.” Clinical Mass Spectrometry 14: 18−30. https://doi.org/10.1016/j.clinms.2019.06.001.
Jain, Nityanand, Tungki Pratama Umar, Anne-Fleur Fahner, and Valdis Gibietis. 2023. “Advancing Therapeutics for Recurrent Clostridioides Difficile Infections: An Overview of Vowst’s FDA Approval and Implications.” Gut Microbes 15 (1): 2232137. https://doi.org/10.1080/19490976.2023.2232137.
Jansson, Janet K., and Erin S. Baker. 2016. “A Multi-omic Future for Microbiome Studies.” Nature Microbiology 1 (5): 16049. https://doi.org/10.1038/nmicrobiol.2016.49.
Jansson, Janet K., and Kirsten S. Hofmockel. 2018. “The Soil Microbiome—From Metagenomics to Metaphenomics.” Current Opinion in Microbiology 43: 162−168. https://doi.org/10.1016/j.mib.2018.01.013.
Jansson, Janet K., and Ruonan Wu. 2023. “Soil Viral Diversity, Ecology and Climate Change.” Nature Reviews Microbiology 21 (5): 296−311. https://doi.org/10.1038/s41579-022-00811-z.
Jeffery, Simon, and Ciro Gardi. 2010. “Soil Biodiversity Under Threat – A Review.” Acta Societatis Zoologicae Bohemicae 74: 7−12.
Johnson, Abigail J., Pajau Vangay, Gabriel A. Al-Ghalith, Benjamin M. Hillmann, Tonya L. Ward, Robin R. Shields-Cutler, Austin D. Kim, Anna Konstantinovna Shmagel, Arzang N. Syed, Jens Walter, Ravi Menon, Katie Koecher, and Dan Knights. 2019. “Daily Sampling Reveals Personalized Diet-Microbiome Associations in Humans.” Cell Host & Microbe 25 (6): 789−802.e5. https://doi.org/10.1016/j.chom.2019.05.005.
Jousset, Alexandre, Christina Bienhold, Antonis Chatzinotas, Laure Gallien, Angélique Gobet, Viola Kurm, Kirsten Küsel, Matthias C. Rillig, Damian W. Rivett, Joana F. Salles, Marcel G. A. van der Heijden, Noha H. Youssef, Xiaowei Zhang, Zhong Wei, and W. H. Gera Hol. 2017. “Where Less May Be More: How the Rare Biosphere Pulls Ecosystems Strings.” The ISME Journal 11 (4): 853−858
Kang, D. W., J. B. Adams, A. C. Gregory, T. Borody, L. Chittick, A. Fasano, A. Khoruts, E. Geis, J. Maldonado, S. McDonough-Means, E. L. Pollard, S. Roux, M. J. Sadowsky, K. S. Lipson, M. B. Sullivan, J. G. Caporaso, and R. Krajmalnik-Brown. 2017. “Microbiota Transfer Therapy Alters Gut Ecosystem and Improves Gastrointestinal and Autism Symptoms: An Open-Label Study.” Microbiome 5 (1): 10. https://doi.org/10.1186/s40168-016-0225-7.
Kaper, James B., James P. Nataro, and Harry L. T. Mobley. 2004. “Pathogenic Escherichia coli.” Nature Reviews Microbiology 2 (2): 123-140. https://doi.org/10.1038/nrmicro818.
Khiyami, Mohammad A., Hassan Almoammar, Yasser M. Awad, Mousa A. Alghuthaymi, and Kamel A. Abd-Elsalam. 2014. “Plant Pathogen Manodiagnostic Techniques: Forthcoming Changes?” Biotechnology & Biotechnological Equipment 28 (5): 775−785. https://doi.org/10.1080/13102818.2014.960739.
King, Dana E., Arch G. Mainous, and Carol A. Lambourne. 2012. “Trends in Dietary Fiber Intake in the United States, 1999-2008.” Journal of the Academy of Nutrition and Dietetics 112 (5): 642−648. https://doi.org/10.1016/j.jand.2012.01.019.
Kishino, Shigenobu, Michiki Takeuchi, Si-Bum Park, Akiko Hirata, Nahoko Kitamura, Jun Kunisawa, Hiroshi Kiyono, Ryo Iwamoto, Yosuke Isobe, Makoto Arita, Hiroyuki Arai, Kazumitsu Ueda, Jun Shima, Satomi Takahashi, Kenzo Yokozeki, Sakayu Shimizu, and Jun Ogawa. 2013. “Polyunsaturated Fatty Acid Saturation by Gut Lactic Acid Bacteria Affecting Host Lipid Composition.” Proceedings of the National Academy of Sciences 110 (44): 17808−17813. https://doi.org/10.1073/pnas.1312937110.
Korem, Tal, David Zeevi, Niv Zmora, Omer Weissbrod, Noam Bar, Maya Lotan-Pompan, Tali Avnit-Sagi, Noa Kosower, Gal Malka, Michal Rein, Jotham Suez, Ben Z. Goldberg, Adina Weinberger, Avraham A. Levy, Eran Elinav, and Eran Segal. 2017. “Bread Affects Clinical Parameters and Induces Gut Microbiome-Associated Personal Glycemic Responses.” Cell Metabolism 25 (6): 1243−1253.e5. https://doi.org/10.1016/j.cmet.2017.05.002.
Larcher, Walter. 1995. Physiological Plant Ecology, 3rd edition. Berlin: Springer-Verlag.
Lauber, Christian L., Kelly S. Ramirez, Zach Aanderud, Jay Lennon, and Noah Fierer. 2013. “Temporal Variability in Soil Microbial Communities Across Land-Use Types.” The ISME Journal 7 (8): 1641−1650. https://doi.org/10.1038/ismej.2013.50.
Le Chatelier, Emmanuelle, Trine Nielsen, Junjie Qin, Edi Prifti, Falk Hildebrand, Gwen Falony, Mathieu Almeida, Manimozhiyan Arumugam, Jean-Michel Batto, Sean Kennedy et al. 2013. “Richness of Human Gut Microbiome Correlates with Metabolic Markers.” Nature 500 (7464): 541−546. https://doi.org/10.1038/nature12506.
Lee, Seung Jae, and Mina Rho. 2022. “Multimodal Deep Learning Applied to Classify Healthy and Disease States of Human Microbiome.” Scientific Reports 12 (1): 824. https://doi.org/10.1038/s41598-022-04773-3.
Leggett, Helen C., Charlie K. Cornwallis, and Stuart A. West. 2012. “Mechanisms of Pathogenesis, Infective Dose and Virulence in Human Parasites.” PLOS Pathogens 8 (2): e1002512. https://doi.org/10.1371/journal.ppat.1002512.
Lehmann, Johannes, Deborah A. Bossio, Ingrid Kögel-Knabner, and Matthias C. Rillig. 2020. “The Concept and Future Prospects of Soil Health.” Nature Reviews Earth & Environment 1 (10): 544−553. https://doi.org/10.1038/s43017-020-0080-8.
Leleiwi, Ikaia, Josué Rodriguez-Ramos, Michael Shaffer, Anice Sabag-Daigle, Katherine Kokkinias, Rory M. Flynn, Rebecca A. Daly, Linnea F. M. Kop, Lindsey M. Solden, Brian M. M. Ahmer, Mikayla A. Borton, and Kelly C. Wrighton. 2023. “Exposing New Taxonomic Variation with Inflammation — A Murine Model-Specific Genome Database for Gut Microbiome Researchers.” Microbiome 11 (1): 114. https://doi.org/10.1186/s40168023-01529-7.
Lennon, Jay T., and Stuart E. Jones. 2011. “Microbial Seed Banks: The Ecological and Evolutionary Implications of Dormancy.” Nature Reviews Microbiology 9 (2): 119−130. https://doi.org/10.1038/nrmicro2504.
Li, Hai, Julien P. Limenitakis, Tobias Fuhrer, Markus B. Geuking, Melissa A. Lawson, Madeleine Wyss, Sandrine Brugiroux, Irene Keller, Jamie A. Macpherson, Sandra Rupp, Bettina Stolp, Jens V. Stein, Bärbel Stecher, Uwe Sauer, Kathy D. McCoy, and Andrew J. Macpherson. 2015. “The Outer Mucus Layer Hosts a Distinct Intestinal Microbial Niche.” Nature Communications 6 (1): 8292. https://doi.org/10.1038/ncomms9292.
Liao, Hu, Hu Li, Chen-Song Duan, Xin-Yuan Zhou, Qiu-Ping Luo, Xin-Li An, Yong-Guan Zhu, and Jian-Qiang Su. 2022. “Response of Soil Viral Communities to Land Use Changes.” Nature Communications 13 (1): 6027. https://doi.org/10.1038/s41467-022-33771-2.
Ling, Ning, Tingting Wang, and Yakov Kuzyakov. 2022. “Rhizosphere Bacteriome Structure and Functions.” Nature Communications 13 (1): 836. https://doi.org/10.1038/s41467022-28448-9.
Lloyd-Price, Jason, Cesar Arze, Ashwin N. Ananthakrishnan, Melanie Schirmer, Julian Avila-Pacheco, Tiffany W. Poon, Elizabeth Andrews, Nadim J. Ajami, Kevin S. Bonham, Colin J. Brislawn, David Casero, Holly Courtney, Antonio Gonzalez, Thomas G. Graeber, A. Brantley Hall, Kathleen Lake, Carol J. Landers, Himel Mallick, Damian R. Plichta, Mahadev Prasad, Gholamali Rahnavard, Jenny Sauk, Dmitry Shungin, Yoshiki Vázquez-Baeza, Richard A. White, Jason Bishai, Kevin Bullock, Amy Deik, Courtney Dennis, Jess L. Kaplan, Hamed Khalili, Lauren J. McIver, Christopher J. Moran, Long Nguyen, Kerry A. Pierce, Randall Schwager, Alexandra Sirota-Madi, Betsy W. Stevens, William Tan, Johanna J. ten Hoeve, George Weingart, Robin G. Wilson, Vijay Yajnik, Jonathan Braun, Lee A. Denson, Janet K. Jansson, Rob Knight, Subra Kugathasan, Dermot P. B. McGovern, Joseph F. Petrosino, Thaddeus S. Stappenbeck, Harland S. Winter, Clary B. Clish, Eric A. Franzosa, Hera Vlamakis, Ramnik J. Xavier, Curtis Huttenhower, and IBDMDB Investigators. 2019. “Multiomics of the Gut Microbial Ecosystem in Inflammatory Bowel Diseases.” Nature 569 (7758): 655−662. https://doi.org/10.1038/s41586-019-1237-9.
Long, Shuping, Yi Yang, Chengpin Shen, Yiwen Wang, Anmei Deng, Qin Qin, and Liang Qiao. 2020. “Metaproteomics Characterizes Human Gut Microbiome Function in Colorectal Cancer.” npj Biofilms and Microbiomes 6 (1): 14. https://doi.org/10.1038/s41522-020-0123-4.
Lozupone, Catherine A., Jesse I. Stombaugh, Jeffrey I. Gordon, Janet K. Jansson, and Rob Knight. 2012. “Diversity, Stability and Resilience of the Human Gut Microbiota.” Nature 489 (7415): 220−230. https://doi.org/10.1038/nature11550.
Lynch, Susan V., and Oluf Pedersen. 2016. “The Human Intestinal Microbiome in Health and Disease.” New England Journal of Medicine 375 (24): 2369−2379. https://doi.org/10.1056/NEJMra1600266.
Ma, Bin, Yiling Wang, Kankan Zhao, Erinne Stirling, Xiaofei Lv, Yijun Yu, Lingfei Hu, Chao Tang, Chuyi Wu, Baiyu Dong, Ran Xue, Randy A. Dahlgren, Xiangfeng Tan, Hengyi Dai, Yong-Guan Zhu, Haiyan Chu, and Jianming Xu. 2024. “Biogeographic Patterns and Drivers of Soil Viromes.” Nature Ecology & Evolution 8 (4): 717−728. https://doi.org/10.1038/s41559-024-02347-2.
Martens, Eric C. 2016. “Fibre for the Future.” Nature 529 (7585): 158−159. https://doi.org/10.1038/529158a.
Martinez, Kristina B., Vanessa Leone, and Eugene B. Chang. 2017. “Microbial Metabolites in Health and Disease: Navigating the Unknown in Search of Function.” Journal of Biological Chemistry 292 (21): 8553−8559. https://doi.org/10.1074/jbc.R116.752899.
Matchado, Monica Steffi, Malte Rühlemann, Sandra Reitmeier, Tim Kacprowski, Fabian Frost, Dirk Haller, Jan Baumbach, and Markus List. 2024. “On the Limits of 16S rRNA Gene-Based Metagenome Prediction and Functional Profiling.” Microbial Genomics 10 (2). https://doi.org/10.1099/mgen.0.001203.
McAnulty, Joanne T., Sharon R. Akabas, Sowmyanarayanan V. Thuppal, Erin E. Paxson, Shilpa Saklani, Katherine L. Tucker, and Regan L. Bailey. 2017. “Fiber Intake Varies by Poverty-Income Ratio and Race/Ethnicity in the US Adults.” Nutrition Today 52 (2): 73−79. https://doi.org/10.1097/nt.0000000000000207.
McGivern, Bridget B., Malak M. Tfaily, Mikayla A. Borton, Suzanne M. Kosina, Rebecca A. Daly, Carrie D. Nicora, Samuel O. Purvine, Allison R. Wong, Mary S. Lipton, David W. Hoyt, Trent R. Northen, Ann E. Hagerman, and Kelly C. Wrighton. 2021. “Decrypting Bacterial Polyphenol Metabolism in an Anoxic Wetland Soil.” Nature Communications 12 (1): 2466. https://doi.org/10.1038/s41467-021-22765-1.
McKeown, Nicola M., Kara A. Livingston, Caleigh M. Sawicki, and Kevin B. Miller. 2020. “Evidence Mapping to Assess the Available Research on Fiber, Whole Grains, and Health.” Nutrition Reviews 78 (Suppl 1): 37−42. https://doi.org/10.1093/nutrit/nuz062.
Nakatsuji, Teruaki, Tiffany H. Chen, Saisindhu Narala, Kimberly A. Chun, Aimee M. Two, Tong Yun, Faiza Shafiq, Paul F. Kotol, Amina Bouslimani, Alexey V. Melnik, Haythem Latif, Ji-Nu Kim, Alexandre Lockhart, Keli Artis, Gloria David, Patricia Taylor, Joanne Streib, Pieter C. Dorrestein, Alex Grier, Steven R. Gill, Karsten Zengler, Tissa R. Hata, Donald Y. M. Leung, and Richard L. Gallo. 2017. “Antimicrobials from Human Skin Commensal Bacteria Protect Against Staphylococcus aureus and Are Deficient in Atopic Dermatitis.” Science Translational Medicine 9 (378). https://doi.org/10.1126/scitranslmed.aah4680.
NASEM (National Academies of Sciences, Engineering, and Medicine). 2017. Microbiomes of the Built Environment: A Research Agenda for Indoor Microbiology, Human Health, and Buildings. Washington, DC: The National Academies Press. https://doi.org/10.17226/23647.
Nguyen, Nhu H., Zewei Song, Scott T. Bates, Sara Branco, Leho Tedersoo, Jon Menke, Jonathan S. Schilling, and Peter G. Kennedy. 2016. “FUNGuild: An Open Annotation Tool for Parsing Fungal Community Datasets by Ecological Guild.” Fungal Ecology 20: 241−248. https://doi.org/10.1016/j.funeco.2015.06.006.
Nguyen, Tra D., Mahbobeh Lesani, Ines Forrest, Yunpeng Lan, Danya A. Dean, Quentin M. R. Gibaut, Yanting Guo, Ekram Hossain, Marcela Olvera, Hannah Panlilio, Adwaita R. Parab, Chaoyi Wu, Jean A. Bernatchez, Robert H. Cichewicz, and Laura-Isobel McCall. 2020. “Local Phenomena Shape Backyard Soil Metabolite Composition.” Metabolites 10 (3): 86. https://www.mdpi.com/2218-1989/10/3/86.
Nicholson, Jeremy K., Elaine Holmes, James Kinross, Remy Burcelin, Glenn Gibson, Wei Jia, and Sven Pettersson. 2012. “Host-Gut Microbiota Metabolic Interactions.” Science 336 (6086): 1262-1267. https://doi.org/ 10.1126/science.1223813.
NMDC (National Microbiome Data Collaborative). 2022. National Microbiome Data Collaborative: Progress Report 2022. U.S. Department of Energy. https://microbiomedata.org/wp-content/uploads/sites/2/2023/03/NMDC-2022-Annual-Report.pdf.
Nogal, Ana, Ana M. Valdes, and Cristina Menni. 2021. “The Role of Short-Chain Fatty Acids in the Interplay Between Gut Microbiota and Diet in Cardio-Metabolic Health.” Gut Microbes 13 (1): 1897212. https://doi.org/10.1080/19490976.2021.1897212.
NRC (National Research Council). 2007. The New Science of Metagenomics: Revealing the Secrets of Our Microbial Planet. Washington, DC: The National Academies Press. https://doi.org/10.17226/11902.
Pasolli, Edoardo, Francesco Asnicar, Serena Manara, Moreno Zolfo, Nicolai Karcher, Federica Armanini, Francesco Beghini, Paolo Manghi, Adrian Tett, Paolo Ghensi, Maria Carmen Collado, Benjamin L. Rice, Casey DuLong, Xochitl C. Morgan, Christopher D. Golden, Christopher Quince, Curtis Huttenhower, and Nicola Segata. 2019. “Extensive Unexplored Human Microbiome Diversity Revealed by Over 150,000 Genomes from Metagenomes Spanning Age, Geography, and Lifestyle.” Cell 176 (3): 649−662.e20. https://doi.org/10.1016/j.cell.2019.01.001.
Penders, John, and Niels van Best. 2022. “The Development of the Gut Microbiota in Childhood and Its Distortion by Lifestyle Changes.” In Evolution, Biodiversity and a Reassessment of the Hygiene Hypothesis, edited by Graham A. W. Rook and Christopher A. Lowry, 197−219. Cham: Springer International Publishing.
Põlme, Sergei, Kessy Abarenkov, R. Henrik Nilsson, Björn D. Lindahl, Karina Engelbrecht Clemmensen, Havard Kauserud, Nhu Nguyen, Rasmus Kjøller, Scott T. Bates, Petr Baldrian et al. 2020. “FungalTraits: A User-Friendly Traits Database of Fungi and Fungus-like Stramenopiles.” Fungal Diversity 105 (1): 1−16. https://doi.org/10.1007/s13225-02000466-2.
Qi, Qian, Caixia Hu, Jiahui Lin, Xuehua Wang, Caixian Tang, Zhongmin Dai, and Jianming Xu. 2022. “Contamination with Multiple Heavy Metals Decreases Microbial Diversity and Favors Generalists as the Keystones in Microbial Occurrence Networks.” Environmental Pollution 306: 119406. https://doi.org/10.1016/j.envpol.2022.119406.
Ramirez, Kelly S., Christopher G. Knight, Mattias de Hollander, Francis Q. Brearley, Bede Constantinides, Anne Cotton, Si Creer, Thomas W. Crowther, John Davison, Manuel Delgado-Baquerizo, Ellen Dorrepaal, David R. Elliott, Graeme Fox, Robert I. Griffiths, Chris Hale, Kyle Hartman, Ashley Houlden, David L. Jones, Eveline J. Krab, Fernando T. Maestre, Krista L. McGuire, Sylvain Monteux, Caroline H. Orr, Wim H. van der Putten, Ian S. Roberts, David A. Robinson, Jennifer D. Rocca, Jennifer Rowntree, Klaus Schlaeppi, Matthew Shepherd, Brajesh K. Singh, Angela L. Straathof, Jennifer M. Bhatnagar, Cécile Thion, Marcel G. A. van der Heijden, and Franciska T. de Vries. 2018. “Detecting Macroecological Patterns in Bacterial Communities Across Independent Studies of Global Soils.” Nature Microbiology 3 (2): 189−196. https://doi.org/10.1038/s41564-017-0062-x.
Ravn, Jonas L., Martin K. M. Engqvist, Johan Larsbrink, and Cecilia Geijer. 2021. “CAZyme Prediction in Ascomycetous Yeast Genomes Guides Discovery of Novel Xylanolytic Species with Diverse Capacities for Hemicellulose Hydrolysis.” Biotechnology for Biofuels 14 (1): 150. https://doi.org/10.1186/s13068-021-01995-x.
Ridaura, Vanessa K., Jeremiah J. Faith, Federico E. Rey, Jiye Cheng, Alexis E. Duncan, Andrew L. Kau, Nicholas W. Griffin, Vincent Lombard, Bernard Henrissat, James R. Bain, Michael J. Muehlbauer, Olga Ilkayeva, Clay F. Semenkovich, Katsuhiko Funai, David K. Hayashi, Barbara J. Lyle, Margaret C. Martini, Luke K. Ursell, Jose C. Clemente, William Van Treuren, William A. Walters, Rob Knight, Christopher B. Newgard, Andrew C. Heath, and Jeffrey I. Gordon. 2013. “Gut Microbiota from Twins Discordant for Obesity Modulate Metabolism in Mice.” Science 341 (6150): 1241214. https://doi.org/10.1126/science.1241214.
Rodríguez-Ramos, Josué A., Mikayla A. Borton, Bridget B. McGivern, Garrett J. Smith, Lindsey M. Solden, Michael Shaffer, Rebecca A. Daly, Samuel O. Purvine, Carrie D. Nicora, Elizabeth K. Eder, Mary Lipton, David W. Hoyt, James C. Stegen, and Kelly C. Wrighton. 2022. “Genome-Resolved Metaproteomics Decodes the Microbial and Viral Contributions to Coupled Carbon and Nitrogen Cycling in River Sediments.” mSystems 7 (4): e00516-22. https://doi.org/10.1128/msystems.00516-22.
Rook, Graham A. W. 2022. “Human Evolution, Microorganisms, Socioeconomic Status and Reconciling Necessary Microbial Exposures with Essential Hygiene.” In Evolution, Biodiversity and a Reassessment of the Hygiene Hypothesis, edited by Graham A. W. Rook and Christopher A. Lowry, 27−66. Cham: Springer International Publishing.
Rook, Graham A. W. 2021. “Darwinian Medicine: We Evolved to Require Continuing Contact with the Microbiota of the Natural Environment. Evolution Turns the Inevitable into a Necessity.” In Microbes: The Foundation Stone of the Biosphere, edited by Christon J. Hurst, 327−364. Cham: Springer International Publishing.
Rook, Graham A. W., and Sally F. Bloomfield. 2021. “Microbial Exposures That Establish Immunoregulation Are Compatible with Targeted Hygiene.” Journal of Allergy and Clinical Immunology 148 (1): 33−39. https://doi.org/10.1016/j.jaci.2021.05.008.
Rook, Graham A. W., Christopher A. Lowry, and Charles L. Raison. 2015. “Hygiene and Other Early Childhood Influences on the Subsequent Function of the Immune System.” Brain Research 1617: 47−62. https://doi.org/10.1016/j.brainres.2014.04.004.
Roux, Simon, and Joanne B. Emerson. 2022. “Diversity in the Soil Virosphere: To Infinity and Beyond?” Trends in Microbiology 30 (11): 1025−1035. https://doi.org/10.1016/j.tim.2022.05.003.
Ryan, Stephanie M., Ramon M. Eichenberger, Roland Ruscher, Paul R. Giacomin, and Alex Loukas. 2020. “Harnessing Helminth-Driven Immunoregulation in the Search for Novel Therapeutic Modalities.” PLOS Pathogens 16 (5): e1008508. https://doi.org/10.1371/journal.ppat.1008508.
Salazar, Alejandro, J. T. Lennon, and J. S. Dukes. 2019. “Microbial Dormancy Improves Predictability of Soil Respiration at the Seasonal Time Scale.” Biogeochemistry 144 (1): 103−116. https://doi.org/10.1007/s10533-019-00574-5.
Santos-Medellin, Christian, Laura A. Zinke, Anneliek M. ter Horst, Danielle L. Gelardi, Sanjai J. Parikh, and Joanne B. Emerson. 2021. “Viromes Outperform Total Metagenomes in Revealing the Spatiotemporal Patterns of Agricultural Soil Viral Communities.” The ISME Journal 15 (7): 1956−1970. https://doi.org/10.1038/s41396-021-00897-y.
Sawicki, Caleigh M., Kara A. Livingston, Martin Obin, Susan B. Roberts, Mei Chung, and Nicola M. McKeown. 2017. “Dietary Fiber and the Human Gut Microbiota: Application of Evidence Mapping Methodology.” Nutrients 9 (2): 125. https://doi.org/10.3390%2Fnu9020125.
Seitz, Valerie A., Bridget B. McGivern, Rebecca A. Daly, Jacqueline M. Chaparro, Mikayla A. Borton, Amy M. Sheflin, Stephen Kresovich, Lindsay Shields, Meagan E. Schipanski, Kelly C. Wrighton, and Jessica E. Prenni. 2022. “Variation in Root Exudate Composition Influences Soil Microbiome Membership and Function.” Applied and Environmental Microbiology 88 (11): e00226-22. https://doi.org/10.1128/aem.00226-22.
Sender, Ron, Shai Fuchs, and Ron Milo. 2016. “Revised Estimates for the Number of Human and Bacteria Cells in the Body.” PLOS Biology 14 (8): e1002533. https://doi.org/10.1371/journal.pbio.1002533.
Shade, Ashley, Stuart E. Jones, J. Gregory Caporaso, Jo Handelsman, Rob Knight, Noah Fierer, and Jack A. Gilbert. 2014. “Conditionally Rare Taxa Disproportionately Contribute to Temporal Changes in Microbial Diversity.” mBio 5 (4). https://doi.org/10.1128/mbio.01371-14.
Sharma, Shikha, Prem Lal Kashyap, and Abhishek Sharma. 2021. “3 - Plant Virome: Current Understanding, Mechanisms, and Role in Phytobiome.” In Microbiomes and Plant Health, edited by Manoj Kumar Solanki, Prem Lal Kashyap, Rizwan Ali Ansari, and Baby Kumari, 53−81. London: Academic Press.
Shi, Shengjing, Erin E. Nuccio, Zhou J. Shi, Zhili He, Jizhong Zhou, and Mary K. Firestone. 2016. “The Interconnected Rhizosphere: High Network Complexity Dominates Rhizosphere Assemblages.” Ecology Letters 19 (8): 926-936. https://doi.org/10.1111/ele.12630.
Šimura, Jan, Ioanna Antoniadi, Jitka Široká, Danu¡e Tarkowská, Miroslav Strnad, Karin Ljung, and Ondřej Novák. 2018. “Plant Hormonomics: Multiple Phytohormone Profiling by Targeted Metabolomics.” Plant Physiology 177 (2): 476−489. https://doi.org/10.1104/pp.18.00293.
Singh, Brajesh K., Hongwei Liu, and Pankaj Trivedi. 2020. “Eco-holobiont: A New Concept to Identify Drivers of Host-associated Microorganisms.” Environmental Microbiology 22 (2): 564−567. https://doi.org/10.1111/1462-2920.14900.
Song, Yang, Shi Yao, Xiaona Li, Tao Wang, Xin Jiang, Nanthi Bolan, Charles R. Warren, Trent R. Northen, and Scott X. Chang. 2024. “Soil Metabolomics: Deciphering Underground Metabolic Webs in Terrestrial Ecosystems.” Eco-Environment & Health 3 (2): 227−237. https://doi.org/10.1016/j.eehl.2024.03.001.
Sparling, G. P. 1997. “Soil Microbial Biomass, Activity and Nutrient Cycling as Indicators of Soil Health.” In Biological Indicators of Soil Health, edited by C. E. Pankhurst, B. M. Doube, and V. V. S. R. Gupta, 97−119. Wallingford: CAB International.
Starke, Robert, Ruebén López Mondéjar, Zander Rainer Human, Diana Navrátilová, Martina Štursová, Tomáš Větrovský, Heather M. Olson, Daniel J. Orton, Stephen J. Callister, Mary S. Lipton, Adina Howe, Lee Ann McCue, Christa Pennacchio, Igor Grigoriev, and Petr Baldrian. 2021. “Niche Differentiation of Bacteria and Fungi in Carbon and Nitrogen Cycling of Different Habitats in a Temperate Coniferous Forest: A Metaproteomic Approach.” Soil Biology and Biochemistry 155: 108170. https://doi.org/10.1016/j.soilbio.2021.108170.
Stott, D. E., S. S. Andrews, M. A. Liebig, B. J. Wienhold, and D. L. Karlen. 2010. “Evaluation of β-Glucosidase Activity as a Soil Quality Indicator for the Soil Management Assessment Framework.” Soil Science Society of America Journal 74 (1): 107−119. https://doi.org/10.2136/sssaj2009.0029.
Strachan, David P. 1989. “Hay Fever, Hygiene, and Household Size.” British Medical Journal 299 (6710): 1259-1260. https://doi.org/10.1136/bmj.299.6710.1259.
Swanson, Jill K., Luis Montes, Luis Mejia, and Caitilyn Allen. 2007. “Detection of Latent Infections of Ralstonia solanacearum Race 3 Biovar 2 in Geranium.” Plant Disease 91 (7): 828−834. https://doi.org/10.1094/pdis-91-7-0828.
Tang, W. H. Wilson, Daniel Y. Li, and Stanley L. Hazen. 2019. “Dietary Metabolism, the Gut Microbiome, and Heart Failure.” Nature Reviews Cardiology 16 (3): 137−154. https://doi.org/10.1038/s41569-018-0108-7.
Thomas, Andrew Maltez, Paolo Manghi, Francesco Asnicar, Edoardo Pasolli, Federica Armanini, Moreno Zolfo, Francesco Beghini, Serena Manara, Nicolai Karcher, Chiara Pozzi, Sara Gandini, Davide Serrano, Sonia Tarallo, Antonio Francavilla, Gaetano Gallo, Mario Trompetto, Giulio Ferrero, Sayaka Mizutani, Hirotsugu Shiroma, Satoshi Shiba, Tatsuhiro Shibata, Shinichi Yachida, Takuji Yamada, Jakob Wirbel, Petra Schrotz-King, Cornelia M. Ulrich, Hermann Brenner, Manimozhiyan Arumugam, Peer Bork, Georg Zeller, Francesca Cordero, Emmanuel Dias-Neto, João Carlos Setubal, Adrian Tett, Barbara Pardini, Maria Rescigno, Levi Waldron, Alessio Naccarati, and Nicola Segata. 2019. “Metagenomic Analysis of Colorectal Cancer Datasets Identifies Cross-cohort Microbial Diagnostic Signatures and a Link with Choline Degradation.” Nature Medicine 25 (4): 667−678. https://doi.org/10.1038/s41591-019-0405-7.
Thompson, Luke R., Jon G. Sanders, Daniel McDonald, Amnon Amir, Joshua Ladau, Kenneth J. Locey, Robert J. Prill, Anupriya Tripathi, Sean M. Gibbons, Gail Ackermann et al. 2017. “A Communal Catalogue Reveals Earth’s Multiscale Microbial Diversity.” Nature 551 (7681): 457−463. https://doi.org/10.1038/nature24621.
Tibbett, Mark, Tandra D. Fraser, and Sarah Duddigan. 2020. “Identifying Potential Threats to Soil Biodiversity.” PeerJ 8: e9271. https://doi.org/10.7717/peerj.9271.
Tindall, Alyssa M., Kristina S. Petersen, and Penny M. Kris-Etherton. 2018. “Dietary Patterns Affect the Gut Microbiome—The Link to Risk of Cardiometabolic Diseases.” Journal of Nutrition 148 (9): 1402−1407. https://doi.org/10.1093/jn/nxy141.
Treseder, Kathleen K., and Jay T. Lennon. 2015. “Fungal Traits That Drive Ecosystem Dynamics on Land.” Microbiology and Molecular Biology Reviews 79 (2): 243−262. https://doi.org/10.1128/mmbr.00001-15.
Trivedi, P., M. Delgado-Baquerizo, C. Trivedi, K. Hamonts, I. C. Anderson, and B. K. Singh. 2017. “Keystone Microbial Taxa Regulate the Invasion of a Fungal Pathogen in Agro-ecosystems.” Soil Biology and Biochemistry 111: 10−14. https://doi.org/10.1016/j.soilbio.2017.03.013.
Turnbaugh, Peter J., Ruth E. Ley, Michael A. Mahowald, Vincent Magrini, Elaine R. Mardis, and Jeffrey I. Gordon. 2006. “An Obesity-Associated Gut Microbiome with Increased Capacity for Energy Harvest.” Nature 444 (7122): 1027−1031. https://doi.org/10.1038/nature05414.
von Mutius, Erika. 2021. “The ‘Hygiene Hypothesis’ and the Lessons Learnt from Farm Studies.” Frontiers in Immunology 12. https://doi.org/10.3389/fimmu.2021.635522.
Wagg, Cameron, Klaus Schlaeppi, Samiran Banerjee, Eiko E. Kuramae, and Marcel G. A. van der Heijden. 2019. “Fungal-Bacterial Diversity and Microbiome Complexity Predict Ecosystem Functioning.” Nature Communications 10 (1): 4841. https://doi.org/10.1038/s41467-019-12798-y.
Wall, Diana H., Uffe N. Nielsen, and Johan Six. 2015. “Soil Biodiversity and Human Health.” Nature 528 (7580): 69−76. https://doi.org/10.1038/nature15744.
Walsh, Corinne M., Isadore Becker-Uncapher, Madeline Carlson, and Noah Fierer. 2021. “Variable Influences of Soil and Seed-Associated Bacterial Communities on the Assembly of Seedling Microbiomes.” The ISME Journal 15 (9): 2748−2762. https://doi.org/10.1038/s41396-021-00967-1.
Wang, Lin, Jia-Bao Cao, Bin-Bin Xia, Yue-Juan Li, Xuan Zhang, Guo-Xin Mo, Rui-Juan Wang, Si-Qi Guo, Yu-Qing Zhang, Kun Xiao, Guang-Fa Zhu, Peng-Fei Liu, Li-Cheng Song, Xi-Hui Ma, Ping-Chao Xiang, Jiang Wang, Yu-Hong Liu, Fei Xie, Xu-Dong Zhang, Xiang-Xin Li, Wan-Lu Sun, Yan Cao, Kai-Fei Wang, Wen-Hui Zhang, Wei-Chao Zhao, Peng Yan, Ji-Chao Chen, Yu-Wei Yang, Zhong-Kuo Yu, Jing-Si Tang, Li Xiao, Jie-Min Zhou, Li-Xin Xie, and Jun Wang. 2023. “Metatranscriptome of Human Lung Microbial Communities in a Cohort of Mechanically Ventilated COVID-19 Omicron Patients.” Signal Transduction and Targeted Therapy 8 (1): 432. https://doi.org/10.1038/s41392-023-01684-1.
Washington, John A. 1996. “Chapter 10 - Principles of Diagnosis.” In Medical Microbiology, 4th edition, edited by Samuel Baron. Galveston, TX: University of Texas Medical Branch at Galveston.
Wassermann, Birgit, Henry Müller, and Gabriele Berg. 2019. “An Apple a Day: Which Bacteria Do We Eat with Organic and Conventional Apples?” Frontiers in Microbiology 10. https://doi.org/10.3389/fmicb.2019.01629.
Wastyk, Hannah C., Gabriela K. Fragiadakis, Dalia Perelman, Dylan Dahan, Bryan D. Merrill, Feiqiao B. Yu, Madeline Topf, Carlos G. Gonzalez, William Van Treuren, Shuo Han, Jennifer L. Robinson, Joshua E. Elias, Erica D. Sonnenburg, Christopher D. Gardner, and Justin L. Sonnenburg. 2021. “Gut-Microbiota-Targeted Diets Modulate Human Immune Status.” Cell 184 (16): 4137−4153.e14. https://doi.org/10.1016/j.cell.2021.06.019.
Weis, Caroline, Aline Cuénod, Bastian Rieck, Olivier Dubuis, Susanne Graf, Claudia Lang, Michael Oberle, Maximilian Brackmann, Kirstine K. Søgaard, Michael Osthoff, Karsten Borgwardt, and Adrian Egli. 2022. “Direct Antimicrobial Resistance Prediction from Clinical MALDI-TOF Mass Spectra Using Machine Learning.” Nature Medicine 28 (1): 164−174. https://doi.org/10.1038/s41591-021-01619-9.
Whidbey, Christopher, and Aaron T. Wright. 2019. “Activity-Based Protein Profiling—Enabling Multimodal Functional Studies of Microbial Communities.” In Activity-Based Protein Profiling, edited by Benjamin F. Cravatt, Ku-Lung Hsu, and Eranthie Weerapana, 1−21. Cham: Springer International Publishing.
Whitman, William B., Tanja Woyke, Hans-Peter Klenk, Yuguang Zhou, Timothy G. Lilburn, Brian J. Beck, Paul De Vos, Peter Vandamme, Jonathan A. Eisen, George Garrity, Philip Hugenholtz, and Nikos C. Kyrpides. 2015. “Genomic Encyclopedia of Bacterial and Archaeal Type Strains, Phase III: The Genomes of Soil and Plant-Associated and Newly Described Type Strains.” Standards in Genomic Sciences 10 (1): 26. https://doi.org/10.1186/s40793-015-0017-x.
Whittaker, Robert J., Katherine J. Willis, and Richard Field. 2001. “Scale and Species Richness: Towards a General, Hierarchical Theory of Species Diversity.” Journal of Biogeography 28 (4): 453−470. https://doi.org/10.1046/j.1365-2699.2001.00563.x.
Wicaksono, Wisnu Adi, Tomislav Cernava, Birgit Wassermann, Ahmed Abdelfattah, Maria J. Soto-Giron, Gerardo V. Toledo, Suvi M. Virtanen, Mikael Knip, Heikki Hyöty, and Gabriele Berg. 2023. “The Edible Plant Microbiome: Evidence for the Occurrence of Fruit and Vegetable Bacteria in the Human Gut.” Gut Microbes 15 (2): 2258565. https://doi.org/10.1080/19490976.2023.2258565.
Wiesmeier, Martin, Frauke Barthold, Benjamin Blank, and Ingrid Kögel-Knabner. 2011. “Digital Mapping of Soil Organic Matter Stocks Using Random Forest Modeling in a Semi-arid Steppe Ecosystem.” Plant and Soil 340 (1): 7−24. https://doi.org/10.1007/s11104-010-0425-z.
Wikoff, William R., Andrew T. Anfora, Jun Liu, Peter G. Schultz, Scott A. Lesley, Eric C. Peters, and Gary Siuzdak. 2009. “Metabolomics Analysis Reveals Large Effects of Gut Microflora on Mammalian Blood Metabolites.” Proceedings of the National Academy of Sciences 106 (10): 3698−3703. https://doi.org/10.1073/pnas.0812874106.
Wilhelm, Roland C., Charles Pepe-Ranney, Pamela Weisenhorn, Mary Lipton, and Daniel H. Buckley. 2021. “Competitive Exclusion and Metabolic Dependency among Microorganisms Structure the Cellulose Economy of an Agricultural Soil.” mBio 12 (1): 10.1128/mbio.03099-20. https://doi.org/ 10.1128/mbio.03099-20.
Wilhelm, Roland C., Harold M. van Es, and Daniel H. Buckley. 2022. “Predicting Measures of Soil Health Using the Microbiome and Supervised Machine Learning.” Soil Biology and Biochemistry 164: 108472. https://doi.org/10.1016/j.soilbio.2021.108472.
Wilhelm, Roland C., Joseph P. Amsili, Kirsten S. M. Kurtz, Harold M. van Es, and Daniel H. Buckley. 2023. “Ecological Insights into Soil Health According to the Genomic Traits and Environment-wide Associations of Bacteria in Agricultural Soils.” ISME Communications 3 (1): 1. https://doi.org/10.1038/s43705-022-00209-1.
Winkler, Thomas W., Felix R. Day, Damien C. Croteau-Chonka, Andrew R. Wood, Adam E. Locke, Reedik Mägi, Teresa Ferreira, Tove Fall, Mariaelisa Graff, Anne E. Justice, Jian’an Luan, Stefan Gustafsson, Joshua C. Randall, Sailaja Vedantam, Tsegaselassie Workalemahu, Tuomas O. Kilpeläinen, André Scherag, Tonu Esko, Zoltán Kutalik, Iris M. Heid, Ruth J. F. Loos, and the Genetic Investigation of Anthropometric Traits Consortium. 2014. “Quality Control and Conduct of Genome-wide Association Meta-analyses.” Nature Protocols 9 (5): 1192−1212. https://doi.org/10.1038/nprot.2014.071.
Withers, Emma, Paul W. Hill, David R. Chadwick, and Davey L. Jones. 2020. “Use of Untargeted Metabolomics for Assessing Soil Quality and Microbial Function.” Soil Biology and Biochemistry 143: 107758. https://doi.org/10.1016/j.soilbio.2020.107758.
Woodcroft, Ben J., Caitlin M. Singleton, Joel A. Boyd, Paul N. Evans, Joanne B. Emerson, Ahmed A. F. Zayed, Robert D. Hoelzle, Timothy O. Lamberton, Carmody K. McCalley, Suzanne B. Hodgkins, Rachel M. Wilson, Samuel O. Purvine, Carrie D. Nicora, Changsheng Li, Steve Frolking, Jeffrey P. Chanton, Patrick M. Crill, Scott R. Saleska, Virginia I. Rich, and Gene W. Tyson. 2018. “Genome-Centric View of Carbon Processing in Thawing Permafrost.” Nature 560 (7716): 49−54. https://doi.org/10.1038/s41586-018-0338-1.
Wrighton, Kelly C., Brian C. Thomas, Itai Sharon, Christopher S. Miller, Cindy J. Castelle, Nathan C. VerBerkmoes, Michael J. Wilkins, Robert L. Hettich, Mary S. Lipton, Kenneth H. Williams, Philip E. Long, and Jillian F. Banfield. 2012. “Fermentation, Hydrogen, and Sulfur Metabolism in Multiple Uncultivated Bacterial Phyla.” Science 337 (6102): 1661−1665. https://doi.org/10.1126/science.1224041.
Wu, Gary D., Jun Chen, Christian Hoffmann, Kyle Bittinger, Ying-Yu Chen, Sue A. Keilbaugh, Meenakshi Bewtra, Dan Knights, William A. Walters, Rob Knight, Rohini Sinha, Erin Gilroy, Kernika Gupta, Robert Baldassano, Lisa Nessel, Hongzhe Li, Frederic D. Bushman, and James D. Lewis. 2011. “Linking Long-Term Dietary Patterns with Gut Microbial Enterotypes.” Science 334 (6052): 105−108. https://doi.org/10.1126/science.1208344.
Yachi, Shigeo, and Michel Loreau. 1999. “Biodiversity and Ecosystem Productivity in a Fluctuating Environment: The Insurance Hypothesis.” Proceedings of the National Academy of Sciences 96 (4): 1463−1468. https://doi.org/10.1073/pnas.96.4.1463.
Yadav, Aanchal, and Rajesh Pandey. 2022. “Viral Infectious Diseases Severity: Co-presence of Transcriptionally Active Microbes (TAMs) Can Play an Integral Role for Disease Severity.” Frontiers in Immunology 13. https://doi.org/10.3389/fimmu.2022.1056036.
Yang, Yi, Yabo Chai, Hanjie Xie, Lu Zhang, Zhiming Zhang, Xue Yang, Shenglei Hao, Jingping Gai, and Yongliang Chen. 2023. “Responses of Soil Microbial Diversity, Network Complexity and Multifunctionality to Three Land-Use Changes.” Science of the Total Environment 859: 160255. https://doi.org/10.1016/j.scitotenv.2022.160255.
Zeevi, David, Tal Korem, Niv Zmora, David Israeli, Daphna Rothschild, Adina Weinberger, Orly Ben-Yacov, Dar Lador, Tali Avnit-Sagi, Maya Lotan-Pompan, Jotham Suez, Jemal Ali Mahdi, Elad Matot, Gal Malka, Noa Kosower, Michal Rein, Gili Zilberman-Schapira, Lenka Dohnalová, Meirav Pevsner-Fischer, Rony Bikovsky, Zamir Halpern, Eran Elinav, and Eran Segal. 2015. “Personalized Nutrition by Prediction of Glycemic Responses.” Cell 163 (5): 1079−1094. https://doi.org/10.1016/j.cell.2015.11.001.
Zhang, Hai-Yang, Andrew Bissett, Carlos A. Aguilar-Trigueros, Hong-Wei Liu, and Jeff R. Powell. 2023. “Fungal Genome Size and Composition Reflect Ecological Strategies Along Soil Fertility Gradients.” Ecology Letters 26 (7): 1108−1118. https://doi.org/10.1111/ele.14224.
Zheng, Danping, Timur Liwinski, and Eran Elinav. 2020. “Interaction Between Microbiota and Immunity in Health and Disease.” Cell Research 30 (6): 492−506. https://doi.org/10.1038/s41422-020-0332-7.
Zheng, Yanfen, Xiaobin Han, Donglin Zhao, Keke Wei, Yuan Yuan, Yiqiang Li, Minghong Liu, and Cheng-Sheng Zhang. 2021. “Exploring Biocontrol Agents from Microbial Keystone Taxa Associated to Suppressive Soil: A New Attempt for a Biocontrol Strategy.” Frontiers in Plant Science 12. https://doi.org/10.3389/fpls.2021.655673.
Zhou, Dongrui, Honglin Zhang, Zhimao Bai, Aidi Zhang, Futian Bai, Xing Luo, Yue Hou, Xiao Ding, Beili Sun, Xiao Sun, Ning Ma, Cuifen Wang, Xiaoniu Dai, and Zuhong Lu. 2016. “Exposure to Soil, House Dust and Decaying Plants Increases Gut Microbial Diversity and Decreases Serum Immunoglobulin E Levels in BALB/c Mice.” Environmental Microbiology 18 (5): 1326−1337. https://doi.org/10.1111/1462-2920.12895.
Zhou, Dongrui, Zhimao Bai, Honglin Zhang, Na Li, Zhiyu Bai, Fudong Cheng, Haitao Jiang, Chuanbin Mao, Xiao Sun, and Zuhong Lu. 2018. “Soil Is a Key Factor Influencing Gut Microbiota and Its Effect Is Comparable to That Exerted by Diet for Mice.” F1000Research 7:1588. https://doi.org/10.12688/f1000research.15297.1.
This page intentionally left blank.